
eBook - ePub
The Inner Solar System
The Sun, Mercury, Venus, Earth, and Mars
Britannica Educational Publishing, Erik Gregersen
This is a test
Share book
- English
- ePUB (mobile friendly)
- Available on iOS & Android
eBook - ePub
The Inner Solar System
The Sun, Mercury, Venus, Earth, and Mars
Britannica Educational Publishing, Erik Gregersen
Book details
Book preview
Table of contents
Citations
About This Book
The planets closest to the SunâMercury, Venus, Earth, and Marsâinclude the world we know and its closest neighbors. However, despite our proximity, these rocky, silicate-based planets still represent so many mysteries yet to discover. Through a trove of images and a narrative bursting with detail, The Inner Solar System imparts what is known about this small corner of the Galaxy, and piques reader interest in the unknown.
Frequently asked questions
How do I cancel my subscription?
Can/how do I download books?
At the moment all of our mobile-responsive ePub books are available to download via the app. Most of our PDFs are also available to download and we're working on making the final remaining ones downloadable now. Learn more here.
What is the difference between the pricing plans?
Both plans give you full access to the library and all of Perlegoâs features. The only differences are the price and subscription period: With the annual plan youâll save around 30% compared to 12 months on the monthly plan.
What is Perlego?
We are an online textbook subscription service, where you can get access to an entire online library for less than the price of a single book per month. With over 1 million books across 1000+ topics, weâve got you covered! Learn more here.
Do you support text-to-speech?
Look out for the read-aloud symbol on your next book to see if you can listen to it. The read-aloud tool reads text aloud for you, highlighting the text as it is being read. You can pause it, speed it up and slow it down. Learn more here.
Is The Inner Solar System an online PDF/ePUB?
Yes, you can access The Inner Solar System by Britannica Educational Publishing, Erik Gregersen in PDF and/or ePUB format, as well as other popular books in Naturwissenschaften & Astronomie & Astrophysik. We have over one million books available in our catalogue for you to explore.
Information
Topic
NaturwissenschaftenSubtopic
Astronomie & AstrophysikCHAPTER 1
COMPOSITION AND ORIGIN OF THE SOLAR SYSTEM
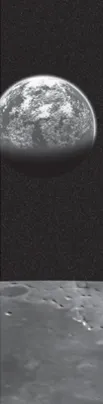
Our home planet, Earth, floats as a tiny oasis of life in the immensity of the universe. However, Earth does not wander space alone. From the earliest ages of history, humanity watched the skies and studied the motions of the Sun, which lit the day, and the Moon, which shone at night. The stars were thought fixed to the vault of heaven, but some stars were wanderers, or planetes, as the Greeks called them. Earth, the Sun, the Moon, and the planets are part of one assembly of nearby astronomical bodies called the solar system.
Located at the centre of the solar system and influencing the motion of all the other bodies through its gravitational force is the Sun, which in itself contains more than 99 percent of the mass of the system. The planets, in order of their distance outward from the Sun, are Mercury, Venus, Earth, Mars, Jupiter, Saturn, Uranus, and Neptune. The first four planets, Mercury, Venus, Earth, and Mars, constitute the inner solar system.
ORBITS
All the planets move around the Sun in elliptical orbits in the same direction that the Sun rotates. This motion is termed prograde, or direct, motion. Looking down on the system from a vantage point above Earthâs North Pole, an observer would find that all these orbital motions are in a counterclockwise direction.
The shape of an objectâs orbit is defined in terms of its eccentricity. For a perfectly circular orbit, the eccentricity is 0; with increasing elongation of the orbitâs shape, the eccentricity increases toward a value of 1, the eccentricity of a parabola. Of the four planets of the inner solar system, Venus has the most circular orbit around the Sun, with an eccentricity of 0.007. Mercury, the closest planet, has the highest eccentricity, with 0.21.
Another defining attribute of an objectâs orbit around the Sun is its inclination, which is the angle that it makes with the plane of Earthâs orbitâthe ecliptic plane. Again, of the inner planets, Mercury has the greatest inclination, its orbit lying at 7° to the ecliptic.
PLANETS AND THEIR MOONS
The eight planets can be divided into two distinct categories on the basis of their densities (mass per unit volume). The four inner, or terrestrial, planetsâMercury, Venus, Earth, and Marsâhave rocky compositions and densities greater than 3 grams per cubic cm (g/cm3; 1.73 oz/in3). (For comparison purposes, water has a density of 1 g/cm3.) In contrast, the four outer planets, also called the Jovian, or giant, planetsâJupiter, Saturn, Uranus, and Neptuneâare large objects with densities less than 2 g/cm3 (1.16 oz/in3).
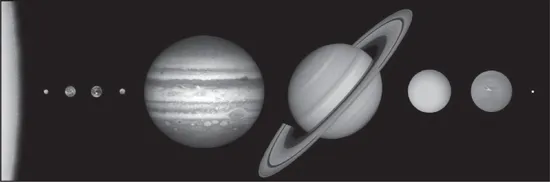
The eight planets of the solar system and dwarf planet Pluto, in a montage of images scaled to show the approximate sizes of the bodies relative to one another. Outward from the Sun, which is represented to scale, are the four rocky terrestrial planets (Mercury, Venus, Earth, and Mars), the four hydrogen-rich giant planets (Jupiter, Saturn, Uranus, and Neptune), and Pluto. NASA/Lunar and Planetary Laboratory.
The relatively small inner planets have solid surfaces, lack ring systems, and have few or no moons. The atmospheres of Venus, Earth, and Mars are composed of a significant percentage of oxidized compounds such as carbon dioxide. Among the inner planets, only Earth has a strong magnetic field, which shields it from the interplanetary medium. The magnetic field traps some of the electrically charged particles of the interplanetary medium inside a region around Earth known as the magnetosphere. Heavy concentrations of these high-energy particles occur in the Van Allen belts in the inner part of the magnetosphere. The moons of Earth and Mars move around their respective planets in the same direction in which those planets orbit the Sun.
THE INTERPLANETARY MEDIUM
The space between the planets of the solar system is not an empty void. It is permeated by thinly scattered matter called the interplanetary medium. The material components of the interplanetary medium consist of neutral hydrogen, the solar wind (discussed in detail in the Sun section), cosmic rays, and dust particles.
Extremely small amounts of neutral (nonionized) hydrogen have been detected throughout much of interplanetary space. At the distance of Earthâs orbit from the Sun, for example, the concentration of neutral hydrogen is about one atom per 100 cubic cm (6 cubic inches). Some of the neutral hydrogen that enters the solar system from interstellar space is ionized by sunlight and by charge exchange with the plasma of the solar wind emanating from the Sun.
Those cosmic rays detected in the vicinity of Earth comprise high-speed, high-energy atomic nuclei and electrons. Among the nuclei, the most abundant are hydrogen nuclei (protons; 90 percent) and helium nuclei (alpha particles; 9 percent). Nuclei outnumber electrons about 50 to 1. A minority of cosmic rays are produced in the Sun, especially at times of increased solar activity. The origin of those coming from outside the solar systemâcalled galactic cosmic raysâremains to be conclusively identified, but they are thought to be produced in stellar processes such as supernova explosions.
Relatively small amounts of dust particles, often called micrometeoroids, exist in the solar system, most of which appear to be orbiting the Sun in or near the plane of the solar system. This dust consists of small grains, generally less than a few hundred micrometres in size and composed of silicate minerals and glassy nodules but sometimes including sulfides, metals, other minerals, and carbonaceous material, in orbit around the Sun. Spacecraft have detected these particles nearly as far out in space as the orbit of Uranus, which indicates that the entire solar system is immersed in a disk of dust, centred on the ecliptic plane.
The existence of interplanetary dust particles was first deduced from observations of zodiacal light, a glowing band visible in the night sky that comprises sunlight scattered by the dust. The zodiacal light is seen in the west after twilight and in the east before dawn, being easily visible in the tropics where the plane of the zodiac, or ecliptic, is approximately vertical. In mid-northern latitudes it is best seen in the evening in February and March and in the morning in September and October. The zodiacal light can be followed visually along the ecliptic from a point 30° from the Sun to about 90°. Photometric measurements indicate that the band continues to the region opposite the Sun where a slight enhancement called the gegenschein, or counterglow, is visible. There is some zodiacal light in all parts of the sky.
Every object in the solar system can produce dust by outgassing, cratering, volcanism, or other processes. Most interplanetary dust is believed to come from the surface erosion and collisions of asteroids, as well as from comets, which give off gas and dust when they travel near the Sun.
The orbits of interplanetary dust particles are easily altered by interaction with the light and charged particles (solar wind) that emanate from the Sun. The smallest particles, less than 0.5 micrometre (0.00002 inch) in size, are blown out of the solar system. Drag effects from sunlight and the solar wind cause larger particles to spiral toward the Sun, some on paths that intercept planets or their moons.
Considered in the context of their collisions with other objects in space, interplanetary dust particles are frequently called micrometeoroids. Because of their high speed (in the tens of kilometres per second), micrometeoroids as small as a few hundred micrometres in size pose a significant collision hazard to spacecraft and their payloads. An impact can, for example, puncture a vital component or create a transient cloud of ions that can short-circuit an electrical system. Consequently, protection against micrometeoroid impacts has become a necessary element of space hardware design. Components of the Earth-orbiting International Space Station use a âdust bumper,â or Whipple shield (named for its inventor, the American astronomer Fred Whipple), to guard against damage from micrometeoroids and orbiting debris. Spacesuits intended for extravehicular activity, which is activity that takes place outside a module or space station, also incorporate micrometeoroid protection in their outer layers.
Analyses of micrometeoroid pitting on Earth-orbiting satellites indicate that about 30,000 tons a year of interplanetary dust strike Earthâs upper atmosphere, mostly particles between 50 ”m and 1 mm (0.002â0.04 inch) in size. Particles from space larger than a few hundred micrometresâi.e., meteoroidsâare heated so severely during deceleration in the atmosphere that they vaporize, producing a glowing meteor trail. Smaller particles experience less severe heating and survive, eventually settling to Earthâs surface. When found in Earthâs atmosphere or on its surface, they are often referred to as micrometeorites or cosmic dust particles.
Using high-altitude research aircraft, the U.S. National Aeronautics and Space Administration (NASA) has collected cosmic dust particles directly from Earthâs stratosphere, where the concentration of terrestrial dust is low. Particles larger than 50 ”m are relatively uncommon there, however, which makes their collection by aircraft impractical. These larger particles have been collected in sediment that has been filtered from large volumes of melted polar ice. Spacecraft missions have been developed to retrieve dust particles directly from space. The U.S. Stardust spacecraft, launched in 1999, flew past Comet Wild 2 in early 2004, collecting particles from its coma (the cloud around a comet formed by evaporating ice) for return to Earth. In 2003 Japanâs space agency launched its Hayabusa spacecraft to return small amounts of surface material, comprising fragments and dust, from the near-Earth asteroid Itokawa for laboratory analysis.
Some cosmic dust particles gathered from the stratosphere are the least altered samples of early solar system dust that have been studied in the laboratory. They provide clues to the temperature, pressure, and chemical composition of the nebular cloud from which the solar system condensed 4.6 billion years ago. The continuous accretion of micrometeorites on early Earth may have contributed organic compounds that were important for the development of life. A few micrometeorites are thought to contain preserved interstellar grainsâsamples of matter from outside the solar system. Spacecraft sample-return missions to comets and asteroids should provide scientists on Earth the opportunity to study even better-preserved material from the birth of the solar system.
There is also a nonmaterial component to the interplanetary medium. The magnetic field lines that are carried outward from the Sun by the solar wind remain attached to the Sunâs surface. Because of the Sunâs rotation, the lines are drawn into a spiral structure. Closely associated with the interplanetary magnetic field are electric forces that act to attract or repel charged particles.
ORIGIN OF THE SOLAR SYSTEM
As the amount of data on the planets, moons, comets, and asteroids has grown, so too have the problems faced by astronomers in forming theories of the origin of the solar system. In the ancient world, theories of the origin of Earth and the objects seen in the sky were certainly much less constrained by fact. Indeed, a scientific approach to the origin of the solar system became possible only after the publication of Isaac Newtonâs laws of motion and gravitation in 1687. Even after this breakthrough, many years elapsed while scientists struggled with applications of Newtonâs laws to explain the apparent motions of planets, moons, comets, and asteroids. Meanwhile, the first semblance of a modern theory was proposed by the German philosopher Immanuel Kant in 1755.
THE KANT-LAPLACE NEBULAR HYPOTHESIS
Kantâs central idea was that the solar system began as a cloud of dispersed particles. He assumed that the mutual gravitational attractions of the particles caused them to start moving and colliding, at which point chemical forces kept them bonded together. As some of these aggregates became larger than others, they grew still more rapidly, ultimately forming the planets. Because Kant was highly versed in neither physics nor mathematics, he did not recognize the intrinsic limitations of his approach. His model does not account for planets moving around the Sun in the same direction and in the same plane, as they are observed to do, nor does it explain the revolution of planetary satellites.
A significant step forward was made by Pierre-Simon Laplace of France some 40 years later. A brilliant mathematician, Laplace was particularly successful in the field of celestial mechanics. Besides publishing a monumental treatise on the subject, he wrote a popular book on astronomy, with an appendix in which he made some suggestions about the origin of the solar system.
Laplaceâs model begins with the Sun already formed and rotating and its atmosphere extending beyond the distance at which the farthest planet would be created. Knowing nothing about the true source of energy in stars, Laplace assumed that the Sun would start to cool as it radiated away its heat. In response to this cooling, as the pressure exerted by its gases declined, the Sun would contract. According to the law of conservation of angular momentum, the decrease in size would be accompanied by an increase in the Sunâs rotational velocity. Centrifugal acceleration would push the material in the atmosphere outward, while gravitational attraction would pull it toward the central mass. When these forces just balanced, a ring of material would be left behind in the plane of the Sunâs equator. This process would have continued through the formation of several concentric rings, each of which then would have coalesced to form a planet. Similarly, a planetâs moons would have originated from rings produced by the forming planets.
Laplaceâs model led naturally to the observed result of planets revolving around the Sun in the same plane and in the same direction as the Sun rotates. Because the theory of Laplace incorporated Kantâs idea of planets coalescing from dispersed material, their two approaches are often combined in a single model called the Kant-Laplace nebular hypothesis. This model for solar system formation was widely accepted for about 100 years. During this period, the apparent regularity of motions in the solar system was contradicted by the discovery of asteroids with highly eccentric orbits and moons with retrograde orbits. Another problem with the nebular hypothesis was the fact that, whereas the Sun contains 99.9 percent of the mass of the solar system, the planets (principally the four giant outer planets) carry more than 99 percent of the systemâs angular momentum. For the solar system to conform to this theory, either the Sun should be rotating more rapidly or the planets should be revolving around it more slowly.
MODERN IDEAS
In the early decades of the 20th century, several scientists decided that the deficiencies of the nebular hypothesis made it no longer tenable. The Americans Thomas Chrowder Chamberlin and Forest Ray Moulton, as well as James Jeans and Harold Jeffreys of Great Britain, independently developed variations on the idea that the planets were formed catastrophicallyâi.e., by a close encounter of the Sun with another star. The basis of this model was that material was drawn out from one or both stars when the two bodies passed at close range, and this material later coalesced to form planets. A discouraging aspect of the theory was the implication that the formation of solar systems in the Milky Way Galaxy must be extremely rare, because sufficiently close encounters between stars would occur very seldom.
The next significant development took place in the mid-20th century as scientists acquired a more mature understanding of the processes by which stars themselves must form and of the behaviour of gases within and around stars. They realized that hot gaseous material stripped from a stellar atmosphere would simply dissipate in space; it would not condense to form planets. Hence, the basic idea that a solar system could form through stellar encounters was untenable. Furthermore, the growth in knowledge about the interstellar medium indicated that large clouds of such matter exist and that stars form in these clouds. Planets must somehow be created in the process that forms the stars themselves. This awareness encouraged scientists to reconsider certain basic processes that resembled some of the earlier notions of Kant and Laplace.
The current approach to the origin of the solar system treats it as part of the general process of star formation. As observational information has steadily increased, the field of plausible models for this process has narrowed. This information ranges from observations of star-forming regions in giant interstellar clouds to subtle clues revealed in the existing chemical composition of the objects present in the solar system. Many scientists have contributed to the modern perspective, most notably the Canadian-born American astrophysicist Alistair G. W. Cameron.
FORMATION OF THE SOLAR NEBULA
The favoured paradigm for the origin of the solar system begins with the gravitational collapse of part of an interstellar cloud of gas and dust having an initial mass only 10 to 20 percent greater than the present mass of the Sun. This type of collapse could be init...