
eBook - ePub
Seed Development and Germination
Jaime Kigel, Jaime Kigel
This is a test
Compartir libro
- 872 páginas
- English
- ePUB (apto para móviles)
- Disponible en iOS y Android
eBook - ePub
Seed Development and Germination
Jaime Kigel, Jaime Kigel
Detalles del libro
Vista previa del libro
Índice
Citas
Información del libro
This text is intended for plant physiologists, molecular biologists, biochemists, biotechnologists, geneticists, horticulturalists, agromnomists and botanists, and upper-level undergraduate and graduate students in these disciplines. It integrates advances in the diverse and rapidly-expanding field of seed science, from ecological and demographic aspects of seed production, dispersal and germination, to the molecular biology of seed development. The book offers a broad, multidisciplinary approach that covers both theoretical and applied knowledge.
Preguntas frecuentes
¿Cómo cancelo mi suscripción?
¿Cómo descargo los libros?
Por el momento, todos nuestros libros ePub adaptables a dispositivos móviles se pueden descargar a través de la aplicación. La mayor parte de nuestros PDF también se puede descargar y ya estamos trabajando para que el resto también sea descargable. Obtén más información aquí.
¿En qué se diferencian los planes de precios?
Ambos planes te permiten acceder por completo a la biblioteca y a todas las funciones de Perlego. Las únicas diferencias son el precio y el período de suscripción: con el plan anual ahorrarás en torno a un 30 % en comparación con 12 meses de un plan mensual.
¿Qué es Perlego?
Somos un servicio de suscripción de libros de texto en línea que te permite acceder a toda una biblioteca en línea por menos de lo que cuesta un libro al mes. Con más de un millón de libros sobre más de 1000 categorías, ¡tenemos todo lo que necesitas! Obtén más información aquí.
¿Perlego ofrece la función de texto a voz?
Busca el símbolo de lectura en voz alta en tu próximo libro para ver si puedes escucharlo. La herramienta de lectura en voz alta lee el texto en voz alta por ti, resaltando el texto a medida que se lee. Puedes pausarla, acelerarla y ralentizarla. Obtén más información aquí.
¿Es Seed Development and Germination un PDF/ePUB en línea?
Sí, puedes acceder a Seed Development and Germination de Jaime Kigel, Jaime Kigel en formato PDF o ePUB, así como a otros libros populares de Scienze biologiche y Botanica. Tenemos más de un millón de libros disponibles en nuestro catálogo para que explores.
Información
10
Acquisition and Loss of Desiccation Tolerance
National Seed Storage Laboratory, USDA-ARS, Fort Collins, Colorado
University of Cape Town, Cape Town, South Africa
I. INTRODUCTION
The ability to withstand complete loss of cellular water is an unusual feature in life; yet it is an adaptive feature of seeds of many species. These seeds are termed “orthodox,” because they can be stored for years under cold dry conditions. Desiccation tolerance is not a universal aspect of seeds. Several plant species from tropical rainforests, temperate forests, and riparian environments produce seeds that are sensitive to desiccation. Because these seeds are difficult to store for more than a single growing season, they are often called “recalcitrant” (Roberts, 1973).
The ability to tolerate desiccation is not limited to seeds, although in spermatophytes, it appears to be more prevalent in the propagating structures such as pollen, seeds, dormant buds, and somatic embryos (Bewley, 1979; Levitt, 1980b; Bewley and Oliver, 1992; Crowe et al., 1992). Desiccation tolerance of vegetative tissues is more common in the lower orders (mosses and algae), and it has been suggested that tolerance in these tissues is based on repair of structures rather than protection, as is hypothesized for seeds (Bewley and Oliver (1992). Despite the widespread occurrence of desiccation tolerance, very little is known about the mechanisms whereby tissues are able to survive dehydration. We could reason that tolerance to desiccation is difficult to achieve and perhaps very energetically costly; otherwise all tissues should be able to adapt to this extreme stress. Vegetative tissues are subject to drying at unpredictable intervals, and thus the mechanisms of tolerance must be readily activated in response to the stress. In contrast, orthodox seeds undergo desiccation in a programmed manner at the termination of their development. Desiccation tolerance is acquired during development of orthodox seeds and it is lost after germination. The purpose of this chapter is to review our understanding of the possible mechanisms of desiccation tolerance in seeds. We will discuss some of the anatomical, biochemical, and biophysical events associated with the onset and loss of desiccation tolerance in orthodox seeds and compare these with developmental aspects of recalcitrant seeds.
II. CHALLENGES OF THE DESICCATED ENVIRONMENT
Water has physical properties that make it an ideal biological solvent; thus it plays many roles in the processes of life. Because it is an incompressible fluid, it can fill cells and organelles, giving them structure. The fluid environment provided by water allows for the diffusion of substrates to the active sites of enzymes. Hydrophilic and hydrophobic interactions stabilize macromolecular conformations and allow for the sequestering of cellular constituents. Water is a reactant or product in many important reactions. Evidence suggests that water may inhibit deleterious reactions by preventing molecules from interacting; in this sense, water also serves as a protectant of macromolecular structure. Because of its myriad roles, water controls the level of metabolic activity in plants (Clegg, 197; Hegarty, 1978; Adams and Rinne, 1980; Mclntyre, 1987; Leopold and Vertucci, 1989). Thus the loss of water will profoundly affect the nature of physical and biochemical reactions (e.g., Karel, 1975; Adams and Rinne, 1980; Leopold and Vertucci, 1989; Bryant and Wolfe, 1992; Crowe et al., 1992). An organism that can tolerate desiccation must be able to prevent, slow down, and/or repair the deleterious reactions induced by the removal of water.
In order to understand the mechanisms of tolerance, it is necessary to understand the nature of damage when water is removed from organisms. We have approached this by reviewing the effects of dehydration at cellular and subcellular levels.
A. Metabolic Stresses
As water is removed from the cell, the concentration of solutes is increased, and eventually the fluidity of the aqueous medium declines. These changes affect the metabolic status of the cell. The changes in metabolic activity are believed to occur at specific moisture levels (Leopold and Vertucci, 1989; Clegg, 1978) (Fig. 1). Critical moisture levels have been postulated for germination metabolism (Palit, 1987), germination (Mclntyre, 1987; Hegarty, 1978; Palit, 1987), continued embryogenesis (Adams and Rinne, 1980; Galau et al., 1991; Rosenberg and Rinne, 1986; Finkelstein and Crouch, 1986; Morris et al., 1991; Fisher et al., 1988; Xu et al., 1990), and the cessation of growth (Adams and Rinne, 1980; Saab and Obendorf, 1989) and cell division (Adams and Rinne, 1980; Myers et al., 1992). Below a moisture level of about −1.5 MPa, tissues no longer grow or expand (e.g., McIntyre, 1987; Hegarty, 1978; Levitt, 1980b), and protein and nucleic acid synthesis patterns change (e.g., Dell’Aquila and Spada, 1992; Dhindsa and Cleland, 1975; Skriver and Mundy, 1990). This slight desiccation may induce production of protectants (Close and Chandler, 1990; Dure et al., 1989; Skriver and Mundy, 1990; Dhindsa, 1991; Chen and Burris, 1990; Blackman et al., 1991, 1992; Bewley, 1979; Kermode, 1990; Bewley and Oliver, 1992). Greater levels of desiccation can result in metabolic imbalances. At about 0.45 g H2O/g dm or about −3 MPa* (according to data from Dell’Aquila, 1992), protein synthesis ceases and repair processes become inoperative (Dhindsa and Cleland, 1975; Osborne, 1983; Dell’Aquila, 1992; Clegg, 1978; Adams and Rinne, 1980). Respiratory activity continues until tissues are dried below about 0.25 g/g or-11 MPa (Leopold and Vertucci, 1989; Vertucci, 1989). At moisture levels between −3 and −11 MPa (about 0.45 to 0.25 g H2O/g dm), catabolic activities continue unabated and processes utilizing the high-energy intermediates are impaired (Leopold and Vertucci, 1989; Vertucci, 1992). These cells may utilize food reserves and accumulate toxins, such as free radicals (LePrince et al., 1990b; 1992; Hendry et al., 1992; McKersie et al., 1988). Desiccation tolerant organisms must be able to withstand such changes in metabolism (Levitt, 1980b; Leprince et al., 1990b, 1992; Rogerson and Matthews, 1977).
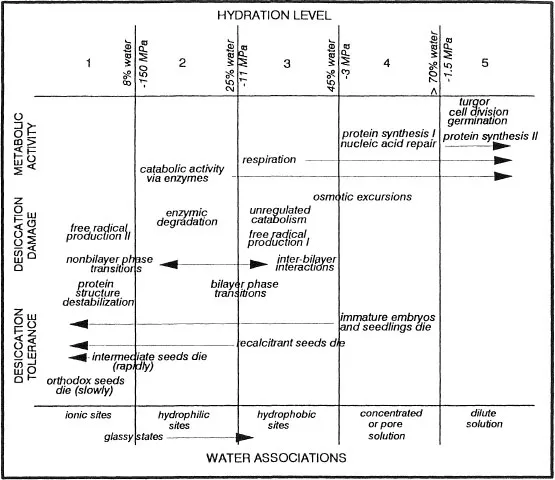
Figure 1 Hydration levels in seeds. There appear to be critical moisture levels at which discrete changes in metabolic activity, damage induced by desiccation, and the properties of water are observed. Here, we present critical moisture levels based on literature cited in this paper. The water potentials given are approximate and require more detailed research, especially for levels 4 and 5. Protein synthesis I and II represent different levels of gene expression. Similarly, free radical production I and II represent different mechanisms of production. The moisture levels for these are also conjectural at this point.
Cells may be more susceptible to the rate of desiccation rather than to loss of water per se (Pritchard, 1991; Pammenter et al., 1991; Berjak et al., 1993; compare Probert and Brierley, 1989 with Kovach and Bradford, 1992; Finch-Savage, 1992a). When held at moisture levels where only catabolic activities occur, cells that are innately desiccation tolerant may suffer damage because they have been subjected to the stress of an unregulated metabolism for a longer time period than if dried rapidly. It is therefore important to realize that the ability to tolerate desiccation must be considered in the context of how the tissue was dried.
B. Mechanical Stresses
One of the first obvious indications of water stress in cells is a loss of turgidity. Under extreme drought, the cells collapse (reviewed by Levitt, 1980a,b). A nonrigid cell wall is required to minimize the tension between the plasmalemma and the cell wall induced by the negative turgor pressure (Iljin’s theory of mechanical stress, reviewed by Levitt, 1980a,b). Cell walls of seed tissues appear to be adapted to accommodate the changes in cell volume (Webb and Arnott, 1982).
The contraction of cells during dehydration can result in the loss of membrane material (reviewed by Steponkus and Webb, 1992, and Hincha and Schmitt, 1992). Meryman (1974) proposed that cells could not contract beyond a “minimum critical volume” without loss of membrane function upon rehydration. Iljin in the 1920s and 1930s (reviewed by Levitt, 1980a,b) observed that cells that were more tolerant of desiccation had only minor reductions in volume when desiccated, and this could be achieved in small cells (about 100 to 1000 μ3), which give relatively large surface-area-to-volume ratios (ca. 1:1) or in cells where accumulated dry matter fills the space previously occupied by water. According to Iljin’s hypothesis of mechanical damage, reduced vacuolar space would also enhance tolerance to desiccation. Furthermore, organelle geometry appears to be an important feature in vegetative tissues possessing desiccation tolerance (Bewley, 1979; Levitt, 1980b; Kaiser, 1982; Oertli, 1986; Bergtrom et al., 1982; Oliver and Bewley, 1984).
During contraction, the plasma membrane vesiculates, and the loss of surface area can result in lysis or liposomes that fail to swell upon subsequent rehydration (Steponkus et al., 1990; Steponkus and Webb, 1992; Hincha and Schmitt, 1992; Johnson-Flanagan and Singh, 1986). The degree of contraction that is tolerated and the amount of membrane material that is irreversibly lost when small vesicles leave the plasmalemma is probably affected by the plasma membrane lipid composition. For example, during acclimation to cold, the phospholipid composition of the plasmalemma changes (Liljenberg and Kates, 1985; Uemura and Steponkus, 1989; Steponkus et al., 1990). Increased cold-hardiness is associated with greater desiccation tolerance (Levitt, 1980a,b), and so one may reason that membrane compositional changes during acclimation to either stress may be comparable. Indeed, increases in the level of unsaturated fatty acids in the phospholipid component is detected during cold acclimation, and addition of these lipid moieties to nonacclimated protoplasts enhances their tolerance to fluctuating water potentials, or “osmotic excursions” (Uemura and Steponkus, 1989). Organisms that are tolerant to desiccation must have a mechanism that allows the reduction of the protoplasmic volume upon dehydration and the subsequent retrieval of the material that may have been lost during vesiculation.
A consequence of protoplasm contraction during dehydration is that the surface-area-to-volume ratio of the protoplast increases. While Iljin’s hypothesis suggests that a relatively high ratio (about 1:1) is associated with desiccation tolerance, theoretical considerations suggest that a very high ratio (such that membranes are within a few nm of each other) contributes to deforming stresses that can alter membrane properties (Wolfe, 1987; Bryant and Wolfe, 1989, 1992). When water is removed from cells, membrane systems become packed together and the interbilayer interactions can cause membrane fusion and/or phase transitions (Lis et al., 1982; Wolfe, 1987; Bryant and Wolfe, 1992; Steponkus and Webb, 1992; Webb and Steponkus, 1993). Organelles with high membrane contents, such as chloroplasts and mitochondria, are particularly susceptible to this type of stress (Wolfe, 1987; Bryant and Wolfe, 1992). Organisms that tolerate dehydration must be able to cope with the mechanical stress resulting from membrane apposition by avoiding the stress (spacers between membranes) or diminishing the strain by optimizing surface-area-to-volume ratios of membranes.
C. Phase Transitions of Lipids
1. Induction by Decreasing Water Content
When moisture levels are considerably reduced, the structure of nucleic acids, proteins, and polar lipids can be altered because the hydrophilic and hydrophobic interactions that stabilize conformations are weakened (Leopold, 1986; Crowe et al., 1987; Carpenter and Crowe, 1988). Membrane systems are considered particularly susceptible to dehydration damage (Crowe et al., 1986, 1987, 1988, 1992; Leopold, 1986; Kerhoas et al., 1987; Wolfe, 1987; Caffrey et al., 1988; McKersie et al., 1988; Bryant and Wolfe, 1989, 1992; Steponkus and Webb, 1992; Quinn, 1985). The losses of membrane function have been attributed to “demixing” (segregation of the various components) of membrane constituents with different hydration character...