
eBook - ePub
Biophotonics for Medical Applications
Igor Meglinski
This is a test
Condividi libro
- 402 pagine
- English
- ePUB (disponibile sull'app)
- Disponibile su iOS e Android
eBook - ePub
Biophotonics for Medical Applications
Igor Meglinski
Dettagli del libro
Anteprima del libro
Indice dei contenuti
Citazioni
Informazioni sul libro
Biophotonics for Medical Applications presents information on the interface between laser optics and cell biology/medicine.
The book discusses the development and application of photonic techniques that aid the diagnosis and therapeutics of biological tissues in both healthy and diseased states. Chapters cover the fundamental technologies used in biophotonics and a wide range of therapeutic and diagnostic applications.
- Presents information on the interface between laser optics and cell biology/medicine
- Discusses the development and application of photonic techniques which aid the diagnosis and therapeutics of biological tissues in both healthy and diseased states
- Presents the fundamental technologies used in biophotonics and a wide range of therapeutic and diagnostic applications
Domande frequenti
Come faccio ad annullare l'abbonamento?
È semplicissimo: basta accedere alla sezione Account nelle Impostazioni e cliccare su "Annulla abbonamento". Dopo la cancellazione, l'abbonamento rimarrà attivo per il periodo rimanente già pagato. Per maggiori informazioni, clicca qui
È possibile scaricare libri? Se sì, come?
Al momento è possibile scaricare tramite l'app tutti i nostri libri ePub mobile-friendly. Anche la maggior parte dei nostri PDF è scaricabile e stiamo lavorando per rendere disponibile quanto prima il download di tutti gli altri file. Per maggiori informazioni, clicca qui
Che differenza c'è tra i piani?
Entrambi i piani ti danno accesso illimitato alla libreria e a tutte le funzionalità di Perlego. Le uniche differenze sono il prezzo e il periodo di abbonamento: con il piano annuale risparmierai circa il 30% rispetto a 12 rate con quello mensile.
Cos'è Perlego?
Perlego è un servizio di abbonamento a testi accademici, che ti permette di accedere a un'intera libreria online a un prezzo inferiore rispetto a quello che pagheresti per acquistare un singolo libro al mese. Con oltre 1 milione di testi suddivisi in più di 1.000 categorie, troverai sicuramente ciò che fa per te! Per maggiori informazioni, clicca qui.
Perlego supporta la sintesi vocale?
Cerca l'icona Sintesi vocale nel prossimo libro che leggerai per verificare se è possibile riprodurre l'audio. Questo strumento permette di leggere il testo a voce alta, evidenziandolo man mano che la lettura procede. Puoi aumentare o diminuire la velocità della sintesi vocale, oppure sospendere la riproduzione. Per maggiori informazioni, clicca qui.
Biophotonics for Medical Applications è disponibile online in formato PDF/ePub?
Sì, puoi accedere a Biophotonics for Medical Applications di Igor Meglinski in formato PDF e/o ePub, così come ad altri libri molto apprezzati nelle sezioni relative a Tecnología e ingeniería e Ingeniería química y bioquímica. Scopri oltre 1 milione di libri disponibili nel nostro catalogo.
Informazioni
Argomento
Tecnología e ingenieríaCategoria
Ingeniería química y bioquímicaPart One
Materials, technologies, and processes
1
Multimodal diffuse optical imaging for biomedical applications
V. Venugopal1; Q. Fang2; X. Intes3 1 Center for Molecular Imaging, Beth Israel Deaconess Medical Center, Harvard Medical School, Boston, MA, USA
2 Martinos Center for Biomedical Imaging, Massachusetts General Hospital, Harvard Medical School, Charlestown, MA, USA
3 Rensselaer Polytechnic Institute, Troy, NY, USA
2 Martinos Center for Biomedical Imaging, Massachusetts General Hospital, Harvard Medical School, Charlestown, MA, USA
3 Rensselaer Polytechnic Institute, Troy, NY, USA
Abstract
Multimodal imaging technologies are increasingly being used in current clinical and preclinical imaging applications owing to the wealth of information provided by the complementary imaging modalities. Optical imaging technologies, which have seen tremendous growth over the last three decades, present a valuable imaging resource for both functional and molecular imaging. In this chapter, we describe efforts toward development of multimodal imaging approaches based on the combination of optical methods with other established imaging modalities like magnetic resonance imaging (MRI), X-ray computed tomography (CT), and ultrasound.
Keywords
Diffuse optical imaging
Optical mammography
Optical-MRI
Optical-CT
Optical-US
Preclinical imaging
1.1 Introduction
Imaging technologies have grown into a central role in current biomedical sciences with significant applications ranging from fundamental medical and biological research to state-of-the-art diagnostic and guidance applications in clinical care. Modalities like magnetic resonance imaging (MRI), X-ray computed tomography (CT), positron emission tomography (PET), and ultrasound (US) have matured over several decades of development and have become the standard of care in present-day hospitals. Multimodal imaging refers to imaging techniques derived from a combination of one or more of the above modalities wherein the complementary nature of information provided by each technique provides the user with a more comprehensive data set (Townsend, 2008). For instance, a functional imaging modality like PET has been combined with structural/anatomical imaging techniques in PET/CT, PET/MRI, and PET/US systems (Pichler et al., 2008). The advantages of such approaches are reflected in the rapid decline in stand-alone PET systems in favor of the multimodality platforms (Townsend, 2008).
Optical imaging of thick tissues is a relatively new imaging modality based on the use of diffused light to probe the functional state of biological tissue (Intes and Chance, 2005b; Gibson et al., 2005). Over the past three decades, the field of optical imaging has grown significantly thanks to the developments in laser and detection instrumentation. Furthermore, the concurrent development in fluorescence-based biomarkers has led to applications of optical imaging methods in molecular imaging (Massoud and Gambhir, 2003; Weissleder and Pittet, 2008). Compared to the aforementioned imaging modalities, optical imaging provides a low-cost solution for medical imaging with high sensitivity and specificity. Today, optical techniques are used in a wide range of applications, including preclinical imaging (Hielscher, 2005), optical mammography (Venugopal and Intes, 2012), and brain imaging (Cutini et al., 2012).
1.1.1 Light interaction with biological tissue
The fundamental principles of optical imaging in thick tissues are governed by two primary phenomena that affect the propagation of photons. The first is scattering of photons due to changes in index of refraction at a microscopic scale which results in the change of direction of propagation. The degree of photon scattering in vivo has been attributed to the nuclear size, cell membranes, and mitochondria (Backman et al., 1999; Beuthan et al., 1996; Beauvoit et al., 1995). Furthermore, the photons are predominantly scattered in the forward direction, scattering as the wavelength of the probing light is appreciably larger than the size of the organelles. The effect of scattering in biological tissues is quantified by the scattering coefficient (μs, unit: cm− 1), which refers to the inverse of distance traveled by the photon before it is scattered (Vo-Dinh, 2003). The second phenomenon affecting photon propagation is the absorption of photons upon transport through tissue. This refers to a loss of energy upon interaction with specific molecules, namely, hemoglobin, water, lipids, melanin, and collagen, which are called chromophores (Woodard and White, 1986). Once again, the degree of energy loss to absorption is dependent on the wavelength of light, and current thick tissue optical imaging methodologies operate in a spectral range that is the most sensitive to oxy-hemoglobin, deoxy-hemoglobin, lipids, and water to probe the functional state of the tissue. More recently, researchers have been incorporating collagen as a functional marker, especially at higher wavelengths (Taroni et al., 2007). Similar to scattering interactions, the absorptive effects of biological tissue is quantified by the absorption coefficient (μa, unit: cm− 1), which indicates the inverse of the average distance traveled by a photon in the tissue before it is absorbed (Vo-Dinh, 2003).
As mentioned above, the interaction of photons in biological samples is highly dependent on the probing wavelength. Figure 1.1 shows the wavelength dependence of scattering and absorption coefficients. It should be noted that the strong absorption and scattering of photons in the ultraviolet and visible wavelength range result in poor propagation of light through the tissue at these wavelengths. Furthermore, it can be deduced that the effect of optical properties are significantly lower for the wavelength range extending from 700 to 900 nm owing to lower absorption by the tissue chromophores. The spectral range referred to as the near-infrared (NIR) window is therefore best suited for optical imaging application and allows deep propagation of photons (as far as 15 cm in the case of breast tissue). Moreover, the spectral dependence of the absorption effects of the tissue chromophores allows us to distinguish and quantify the relative concentration of different chromophores by probing the tissue at different wavelengths. This constitutes the fundamental approach to functional imaging using optical methods wherein the relative concentrations can provide valuable information regarding the functional state of the tissue.
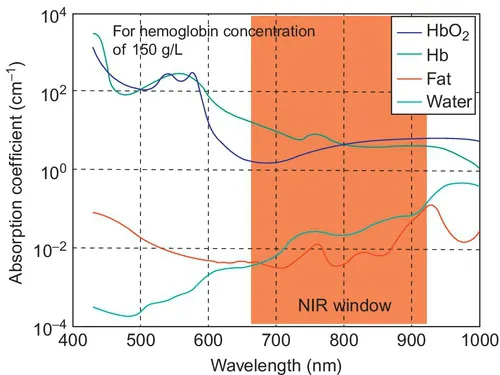
Figure 1.1 Absorption spectra of primary chromophores in biological tissue.
Another phenomenon commonly encountered in optical imaging is fluorescence. Fluorescence is an absorption-mediated phenomenon wherein the molecular species absorbing the photon releases a lower-energy photon (at a different wavelength) (Lakowicz, 2006). The fluorescence signal thus observed is characterized by two parameters. First is the quantum yield, which refers to the efficiency of photon generation upon absorption. The second parameter is the half-life of fluorescence decay, which is also referred to as lifetime. Both these parameters are sensitive to tissue microenvironment conditions (e.g., pH, temperature, etc.) and are thus used in current optical imaging method to obtain sensitive information about the molecular state of the tissue (Lakowicz, 2006). Several endogenous molecules, such as NaDH, have been used for fluorescence optical imaging (Mycek and Pogue, 2003). However, endogenous fluorophores typically operate in the ultraviolet and visible ranges and are therefore not suitable for thick tissue imaging. Conversely, there has been significant interest in recent years in the development of exogenous dyes that operate in the NIR window (Licha, 2002). Concurrently, several fluorescence imaging applications have grown owing to the high detectability of NIR dyes in thick tissue for both preclinical and clinical imaging applications. Furthermore, specialized dyes with controllable fluorescence properties (based on the microenvironment conditions) have established optical methods at the forefront of molecular imaging research (Licha et al., 2012).
1.1.2 Multimodal approaches in optical imaging
As mentioned in the previous section, typical multimodal imaging modalities are based upon the combination of a functional imaging technique with a high-resolution structural imaging modality to provide more comprehensive information. Furthermore, PET imaging is the standard of care in functional imaging; however, it has its drawbacks in the use of ionizing radiation and the need for relatively expensive e...