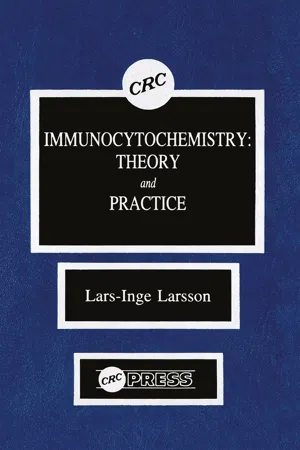
eBook - ePub
Immunocytochemistry
Theory and Practice
Lars-Inge Larsson
This is a test
- 288 pagine
- English
- ePUB (disponibile sull'app)
- Disponibile su iOS e Android
eBook - ePub
Immunocytochemistry
Theory and Practice
Lars-Inge Larsson
Dettagli del libro
Anteprima del libro
Indice dei contenuti
Citazioni
Informazioni sul libro
A complete and balanced overview of all aspects of immunocytochemistry is presented providing a clear understanding of their impact on experiment. All available techniques and many diagnostic and research applications are included, as well as practical step-by-step instructions for carrying out recommended methods. Intended for the novice as well as the experienced researchers.
Domande frequenti
Come faccio ad annullare l'abbonamento?
È semplicissimo: basta accedere alla sezione Account nelle Impostazioni e cliccare su "Annulla abbonamento". Dopo la cancellazione, l'abbonamento rimarrà attivo per il periodo rimanente già pagato. Per maggiori informazioni, clicca qui
È possibile scaricare libri? Se sì, come?
Al momento è possibile scaricare tramite l'app tutti i nostri libri ePub mobile-friendly. Anche la maggior parte dei nostri PDF è scaricabile e stiamo lavorando per rendere disponibile quanto prima il download di tutti gli altri file. Per maggiori informazioni, clicca qui
Che differenza c'è tra i piani?
Entrambi i piani ti danno accesso illimitato alla libreria e a tutte le funzionalità di Perlego. Le uniche differenze sono il prezzo e il periodo di abbonamento: con il piano annuale risparmierai circa il 30% rispetto a 12 rate con quello mensile.
Cos'è Perlego?
Perlego è un servizio di abbonamento a testi accademici, che ti permette di accedere a un'intera libreria online a un prezzo inferiore rispetto a quello che pagheresti per acquistare un singolo libro al mese. Con oltre 1 milione di testi suddivisi in più di 1.000 categorie, troverai sicuramente ciò che fa per te! Per maggiori informazioni, clicca qui.
Perlego supporta la sintesi vocale?
Cerca l'icona Sintesi vocale nel prossimo libro che leggerai per verificare se è possibile riprodurre l'audio. Questo strumento permette di leggere il testo a voce alta, evidenziandolo man mano che la lettura procede. Puoi aumentare o diminuire la velocità della sintesi vocale, oppure sospendere la riproduzione. Per maggiori informazioni, clicca qui.
Immunocytochemistry è disponibile online in formato PDF/ePub?
Sì, puoi accedere a Immunocytochemistry di Lars-Inge Larsson in formato PDF e/o ePub, così come ad altri libri molto apprezzati nelle sezioni relative a Médecine e Théorie, pratique et référence de la médecine. Scopri oltre 1 milione di libri disponibili nel nostro catalogo.
Informazioni
Chapter 1
ANTIBODIES AND ANTISERA
1. GENERAL ASPECTS OF ANTIBODIES AND ANTIGEN-ANTIBODY INTERACTIONS
Nearly all antibodies used in immunocytochemistry and related techniques belong to the immunoglobulin G (IgG) class, the remainder being IgM antibodies. IgG molecules have an average size of 4 x 5 x 8 nm and a molecular weight of 160,000 daltons.45 They are glycoproteins and consist of two identical Fab regions and one Fc fragment. The antibody-combining (antigen-binding) sites are localized in the Fab regions, which have a variable amino acid composition. The rest of the IgG molecule has a constant amino acid composition for each subclass. Recent studies have revealed that the binding site shows less structural variability than previously supposed, and a model of its geometry has been devised as a nine-stranded cylindrical barrel with an average radius of 0.84 nm.46 The Fab region of each IgG molecule is built up by a light (L) and a heavy (H) chain, whereas the crystalline fragment (Fc) is built up by heavy chains alone (Figure 1). The Fc fragment has no antigen-binding capacity, but binds a large number of different molecules, including complement components and protein A. As will be seen this capacity has been exploited in immunocytochemical techniques (complement-binding techniques, labeled protein A methods). At least some IgG molecules appear to be capable of binding protein A also with their Fab region (cf. Chapter 3, Section II.C). The Fc region is not crucial for antigen binding. Digestion of IgG with pepsin splits the molecule into a pFc′ fragment and an F(ab′)2 fragment (Figure 1). Mild reduction of the F(ab′)2 fragment releases the individual F(ab′) fragments. F(ab′)2 and Fab fragments have been employed in immunocytochemistry in cases where penetration into tissues is important (particularly in pre-embedding staining). F(ab′) fragments can be made to rejoin and reform the disulfide bridges. Importantly, such reformation also occurs between F(ab′) fragments with different specificities, making production of hybrid antibodies feasible. Thus, F(ab′)2 fragments consisting of, e.g., one marker (e.g., ferritin or enzyme) binding site and one site binding the antigen of interest can be fabricated.

It is evident that the size of the antigen-combining site will be smaller than that of most antigens. Consequently, it will bind only to a part of the antigen. It is a common misconception that the immunologist by his immunization scheme “instructs” the immunized animal to produce antibodies against a particular chosen antigen. Rather, according to modern immunological theory, introduction of a foreign substance stimulates the proliferation of, and antibody formation by, a particular subset of lymphocytes producing antibodies with the best fit against the substance. In a mouse, the total antibody repertoire is estimated to exceed more than 108 (see Reference 29). Nevertheless, this figure indicates that many antigenic determinants (or epitopes) in antigens are similar. Recent data obtained by Westhof et al.83 and Tainer et al.71 have indicated that antibodies preferentially bind to protein regions with a high degree of mobility or flexibility. Westhof et al.83 suggested that the mobility of the antigenic region may make it easier for an antigen to bind to an antibody not preconceived by nature to bind it. Thus, the antigen does not instruct the production of a perfectly fitting antibody, but selects clones of antibody-forming cells with the best possible fit. It seems reasonable that highly mobile regions of antigens stand a better chance of selecting a clone producing antibodies with a good fit. Together, these data indicate that the interactions between antigens and antibodies are dynamic and not static (as in the classical key-lock model). Nevertheless, during successful immunizations antibodies with very high binding energies (109 to 1012 ℓ/mol) are produced in large quantity. Such binding energies are strong enough to withstand dissociation under most nondenaturing conditions. This is one of the reasons why antibody elution techniques and affinity purification schemes work only with low- to medium-avidity antibodies. In polyclonal antisera, antibody subpopulations of different specificities and different avidities occur. During antigen-antibody incubation, low-avidity antibodies may bind to and block epitopes that otherwise would have bound high-avidity antibodies. During subsequent washings, low-avidity antibodies become dislodged and do not contribute to staining. By repeating the antiserum incubation, epitopes freed of low-avidity antibodies during washings stand a renewed chance of binding high-avidity antibodies. In immunocytochemistry, such repeated application of antibodies has been shown to result in increased detection efficiency and also in improved absolute sensitivity.26,60 Simple as it may be, this observation has proven very important both for pressing sensitivity and for increasing dilutions (and decreasing cost) of antibodies. As expected from the above considerations, prolonged and/or repeated antibody incubation is beneficial to a variable degree with different primary antibodies but is of little help with monoclonal antibodies which already consist of only one antibody species having only one avidity.
Experiments with synthetic peptides, as well as with protein antigens, reveal that the antigenic site or epitope is very small indeed and usually consists of only three to eight amino acid residues. These residues may form part of a continuous stretch of a peptide sequence (continuous antigenic site). In other cases they may be separated from each other in the primary protein sequence, but may be brought together in the native folded protein by the protein conformation (discontinuous antigenic site).1,2 It has proven possible to synthesize short peptides that mimic the antigenic sites of proteins. In cases of discontinuous antigenic sites such synthetic peptides may include “spacer amino acids” to fill the gap between the residues brought together by conformation. In this way, antigenic sites in several proteins have been mapped and identified.2 A somewhat different approach has been taken by Lerner’s group, which has used small synthetic peptides as antigens to elicit antibodies that can react with large proteins incorporating the peptide sequence.41,69,71 Interestingly, not only known antigenic regions in the whole protein, but also regions that would not have elicited immune responses in animals injected with the whole protein may be reactive with antisynthetic peptide antibodies. Again, antibodies made to highly mobile peptide regions react well with the native protein, whereas those against well-ordered regions do not.71 This approach holds considerable promise for many avenues of research, including the development of synthetic vaccines and production of antibodies to proteins where only the DNA or RNA sequence is known.41
As antibodies to peptides and proteins recognize a stretch of only three to eight amino acids, cross-reactivity phenomena are difficult to avoid. Assuming that 20 amino acids can be used to build peptides and proteins, a tripeptide unit can be made in 8,000 (203) ways, a tetrapeptide unit can be made in 160,000 (204) ways, etc. Thus, from purely statistical reasoning, cross-reactivity phenomena can be expected with high frequency. However, the natural amino acids may be post-translationally modified, e.g., by phosphorylation, sulfation, amidation, and acetylation. Moreover, the presence of certain amino acids at the free N- or C-terminal ends are often required by peptide antibodies. In addition, even though they are not themselves an integral part of the antigenic site, certain amino acids surrounding this site may influence the conformation and overall charge of the epitope and thereby promote or reduce its antibody binding. Together, these factors tend to somewhat reduce the great probability of cross-reactivity. Nevertheless, cross-reactivity phenomena are very frequently encountered. One of the reasons for this is that certain amino acid regions have been strongly conserved during evolution. One example is oncogene proteins, of which many contain a characteristic site for tyrosine phosphorylation. Not only is this site repeated in many oncogenes, it is also found in some growth factor receptors and in several peptide hormones such as gastrin and human growth hormone (Figure 2). Not all of these proteins appear to be tyrosine phosphorylated but their sequence similarities are sufficiently great to allow certain antibodies to cross-react. Peptide hormones often share substantial amino acid homologies, allowing them to be grouped into hormone families. However, substantial homologies occur also between families. Some such homologies, referred to as “sliding homologies”, between hormones, oncogenes, growth factors, receptors, and other proteins are illustrated in Figure 2. Immunocytochemical research into peptide hormones and neuropeptides has indeed revealed numerous examples of cross-reactivity phenomena often leading to major misinterpretations of experimental data. Antisera made to a synthetic peptide (src-c) of the Rous sarcoma virus-transforming protein were also found to cross-react with a number of proteins in normal untransformed cells.44 These cross-reacting proteins included inter alia (i.a.) the cytoskeletal proteins tubulin, myosin, and vimentin.44 From a theoretical point of view, one would expect monoclonal antibodies to be less prone to cross-reactivity phenomena than polyclonal antisera. Hence, the latter contain many subpopulations of antibodies with a variable degree of “fit” between epitope and combining site. Thus, it is frequently observed that monoclonal antibodies are less prone to reveal interspecies cross-reactivity between related proteins. However, the theoretical considerations discussed above still prevail, and in practice, many examples of monoclonal antibody cross-reactivities have been gathered. Thus, Horejsí and Dráber have described a monoclonal tubulin antibody that cross-reacts with human serum albumin.27 Moreover, cross-reactivity of monoclonal met-enkephalin antibodies to cholecystokinin (CCK) has been detected, and widespread reactivity of monoclonal antibodies with numerous tissues has been found.13,57,58 It is not possible to prove that an antibody does not cross-react with other proteins. Thus most proteins have still to be isolated and sequenced, and even if they were, cross-reactivity tests against them would be impractical. Immunodiffusion, immunoelectrophoresis, and, particularly, immunoblotting techniques are frequently cited as proofs for antibody “monospecificity”. However, the first two techniques have a sensivity far below that of immunocytochemistry. The third method, immunoblotting, is restricted to antigens that can be solubilized, electrophoretically resolved, and successfully blotted onto filters. First, not all proteins are solubilized and will not enter the gel. Second, some smaller antigens will not be resolved on the gel or will move out of the gel due to their low molecular weight. Third, not all antigens are quantitatively blotted over onto the filter paper, and the blotting conditions and blotting buffer have major impacts on transfer of different proteins. Finally, in most applications, proteins may be severely denatured during electrophoresis (heat, SDS, or mercaptoethanol), and during blotting. Thus, immunoblotting is a powerful technique to prove that the protein sought is present i...
Indice dei contenuti
Stili delle citazioni per Immunocytochemistry
APA 6 Citation
Larsson, L.-I. (2020). Immunocytochemistry (1st ed.). CRC Press. Retrieved from https://www.perlego.com/book/2011679/immunocytochemistry-theory-and-practice-pdf (Original work published 2020)
Chicago Citation
Larsson, Lars-Inge. (2020) 2020. Immunocytochemistry. 1st ed. CRC Press. https://www.perlego.com/book/2011679/immunocytochemistry-theory-and-practice-pdf.
Harvard Citation
Larsson, L.-I. (2020) Immunocytochemistry. 1st edn. CRC Press. Available at: https://www.perlego.com/book/2011679/immunocytochemistry-theory-and-practice-pdf (Accessed: 15 October 2022).
MLA 7 Citation
Larsson, Lars-Inge. Immunocytochemistry. 1st ed. CRC Press, 2020. Web. 15 Oct. 2022.