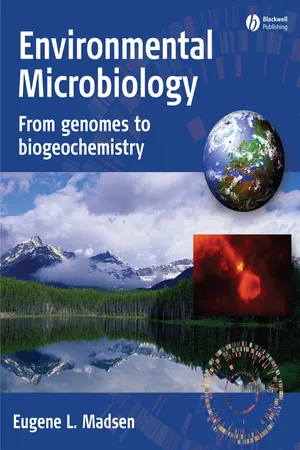
eBook - ePub
Environmental Microbiology
From Genomes to Biogeochemistry
Eugene L. Madsen
This is a test
Share book
- English
- ePUB (mobile friendly)
- Available on iOS & Android
eBook - ePub
Environmental Microbiology
From Genomes to Biogeochemistry
Eugene L. Madsen
Book details
Book preview
Table of contents
Citations
About This Book
This well-referenced, inquiry-driven text presents an up-to-date and comprehensive understanding of the emerging field of environmental microbiology.
- Coherent and comprehensive treatment of the dynamic, emerging field of environmental microbiology
- Emphasis on real-world habitats and selective pressures experienced by naturally occurring microorganisms
- Case studies and "Science and the Citizen" features relate issues in the public's mind to the underlying science
- Unique emphasis on current methodologies and strategies for conducting environmental microbiological research, including methods, logic, and data interpretation
Frequently asked questions
How do I cancel my subscription?
Can/how do I download books?
At the moment all of our mobile-responsive ePub books are available to download via the app. Most of our PDFs are also available to download and we're working on making the final remaining ones downloadable now. Learn more here.
What is the difference between the pricing plans?
Both plans give you full access to the library and all of Perlegoâs features. The only differences are the price and subscription period: With the annual plan youâll save around 30% compared to 12 months on the monthly plan.
What is Perlego?
We are an online textbook subscription service, where you can get access to an entire online library for less than the price of a single book per month. With over 1 million books across 1000+ topics, weâve got you covered! Learn more here.
Do you support text-to-speech?
Look out for the read-aloud symbol on your next book to see if you can listen to it. The read-aloud tool reads text aloud for you, highlighting the text as it is being read. You can pause it, speed it up and slow it down. Learn more here.
Is Environmental Microbiology an online PDF/ePUB?
Yes, you can access Environmental Microbiology by Eugene L. Madsen in PDF and/or ePUB format, as well as other popular books in Sciences biologiques & Microbiologie. We have over one million books available in our catalogue for you to explore.
Information
1
Significance, History, and Challenges of Environmental Microbiology
This chapter is designed to instill in the reader a sense of the goals, scope, and excitement that permeate the discipline of environmental microbiology. We begin with five core concepts that unify the field. These are strengthened and expanded throughout the book. Next, an overview of the significance of environmental microbiology is presented, followed by a synopsis of key scholarly events contributing to environmental microbiology's rich heritage. The chapter closes by reminding the reader of the complexity of Earth's biogeochemical systems and that strategies integrating information from many scientific disciplines can improve our understanding of biosphere function.
Chapter 1 Outline
1.1 Core concepts can unify environmental microbiology
1.2 Synopsis of the significance of environmental microbiology
1.3 A brief history of environmental microbiology
1.4 Complexity of our world
1.5 Many disciplines and their integration
1.1 CORE CONCEPTS CAN UNIFY ENVIRONMENTAL MICROBIOLOGY
Environmental microbiology is inherently multidisciplinary. Its many disparate areas of science need to be presented coherently. To work toward that synthesis, this text uses five recurrent core concepts to bind and organize facts and ideas.
Core concept 1. Environmental microbiology is like a child's picture of a house - it has (at least) five sides (a floor, two vertical sides, and two sloping roof pieces). The floor is evolution. The walls are thermodynamics and habitat diversity. The roof pieces are ecology and physiology. To learn environmental microbiology we must master and unite all sides of the house.
Core concept 2. The prime directive for microbial life is survival, maintenance, generation of adenosine triphosphate (ATP), and sporadic growth (generation of new cells). To predict and understand microbial processes in real-world waters, soils, sediments, and other habitats, it is helpful to keep the prime directive in mind.
Core concept 3. There is a mechanistic series of linkages between our planet's habitat diversity and what is recorded in the genomes of microorganisms found in the world today. Diversity in habitats is synonymous with diversity in selective pressures and resources. When operated upon by forces of evolution, the result is molecular, metabolic, and physiological diversity found in extant microorganisms and recorded in their genomes.
Core concept 4. Advancements in environmental microbiology depend upon convergent lines of independent evidence using many measurement procedures. These include microscopy, biomarkers, model cultivated microorganisms, molecular biology, and genomic techniques applied to laboratory and field-based investigations.
Core concept 5. Environmental microbiology is a dynamic, methods limited discipline. Each methodology used by environmental microbiologists has its own set of strengths, weaknesses, and potential artifacts. As new methodologies deliver new types of information to environmental microbiology, practitioners need a sound foundation that affords interpretation of the meaning and place of the incoming discoveries.
1.2 SYNOPSIS OF THE SIGNIFICANCE OF ENVIRONMENTAL MICROBIOLOGY
With the formation of planet Earth 4.6 Ă 109 years ago, an uncharted series of physical, chemical, biochemical, and (later) biological events began to unfold. Many of these events were slow or random or improbable. Regardless of the precise details of how life developed on Earth, (see Sections 2.3â2.7), it is now clear that for ~70% of life's history, prokaryotes were the sole or dominant life forms. Prokaryotes (Bacteria and Archaea) were (and remain) not just witnesses of geologic, atmospheric, geochemical, and climatic changes that have occurred over the eons. Prokaryotes are also active participants and causative agents of many geochemical reactions found in the geologic record. Admittedly, modern eukaryotes (especially land plants) have been major biogeochemical and ecological players on planet Earth during the most recent 1.4 Ă 109 years. Nonetheless, today, as always, prokaryotes remain the âhostsâ of the planet. Prokaryotes comprise ~60% of the total biomass (Whitman et al., 1998; see Chapter 4), account for as much as 60% of total respiration of some terrestrial habitats (Velvis, 1997; Hanson et al., 2000), and also colonize a variety of Earth's habitats devoid of eukaryotic life due to topographic, climatic and geochemical extremes of elevation, depth, pressure, pH, salinity, heat, or light.
The Earth's habitats present complex gradients of environmental conditions that include variations in temperature, light, pH, pressure, salinity, and both inorganic and organic compounds. The inorganic materials range from elemental sulfur to ammonia, hydrogen gas, and methane and the organic materials range from cellulose to lignin, fats, proteins, lipids, nucleic acid, and humic substances (see Chapter 7). Each geochemical setting (e.g., anaerobic peatlands, oceanic hydrothermal vents, soil humus, deep subsurface sediments) features its own set of resources that can be physiologically exploited by microorganisms. The thermodynamically governed interactions between these resources, their settings, microorganisms themselves, and 3.6 Ă 109 years of evolution are probably the source of metabolic diversity of the microbial world.
Table 1.1 Microorganisms' unique combination of traits and their broad impact on the biosphere
Traits of microorganisms | Ecological consequences of traits |
Small size | Geochemical cycling of elements |
Ubiquitous distribution throughout Earth's habitats | Detoxification of organic pollutants |
High specific surface areas | Detoxification of inorganic pollutants |
Potentially high rate of metabolic activity | Release of essential limiting nutrients from the biomass in one generation to the next |
Physiological responsiveness | |
Genetic malleability | Maintaining the chemical composition of soil sediment, water, and atmosphere required by other forms of life Unrivaled enzymatic diversity |
Potential rapid growth rate | |
Unrivaled nutritional diversity | |
Unrivaled enzymatic diversity |
Microorganisms are the primary agents of geochemical change. Their unique combination of traits (Table 1.1) cast microorganisms in the role of recycling agents for the biosphere. Enzymes accelerate reaction rates between thermodynamically unstable substances. Perhaps the most ecologically important types of enzymatic reactions are those that catalyze oxidation/reduction reactions between electron donors and electron acceptors. These allow microorganisms to generate metabolic energy, survive, and grow. Microorganisms procreate by carrying out complex, genetically regulated sequences of biosynthetic and assimilative intracellular processes. Each daughter cell has essentially the same macro-molecular and elemental composition as its parent. Thus, integrated metabolism of all nutrients (e.g., carbon, nitrogen, phosphorus, sulfur, oxygen, hydrogen, etc.) is implicit in microbial growth. This growth and survival of microorganisms drives the geochemical cycling of the elements, detoxifies many contaminant organic and inorganic compounds, makes essential nutrients present in the biomass of one generation available to the next, and maintains the conditions required by other inhabitants of the biosphere (Table 1.1). Processes carried out by microorganisms in soils, sediments, oceans, lakes, and groundwaters have a major impact on environmental quality, agriculture, and global climate change. These processes are also the basis for current and emerging biotechnologies with industrial and environmental applications (see Chapter 8). Table 1.2 presents a sampling of the ecological and biogeochemical processes that microorganisms catalyze in aquatic or terrestrial habitats. Additional details of biogeochemical processes and ways to recognize and understand them are presented in Chapters 3 and 7.
Table 1.2 Examples of nutrient cycling and physiological processes catalyzed by microorganisms in biosphere habitats (reproduced with permission from Nature Reviews Microbiology from Madsen, E.L. 2005. Identifying microorganisms responsible for ecologically significant biogeochemical processes. Nature Rev. Microbiol. 3:439â446. Macmillan Magazines, http://www.nature.com/reviews)
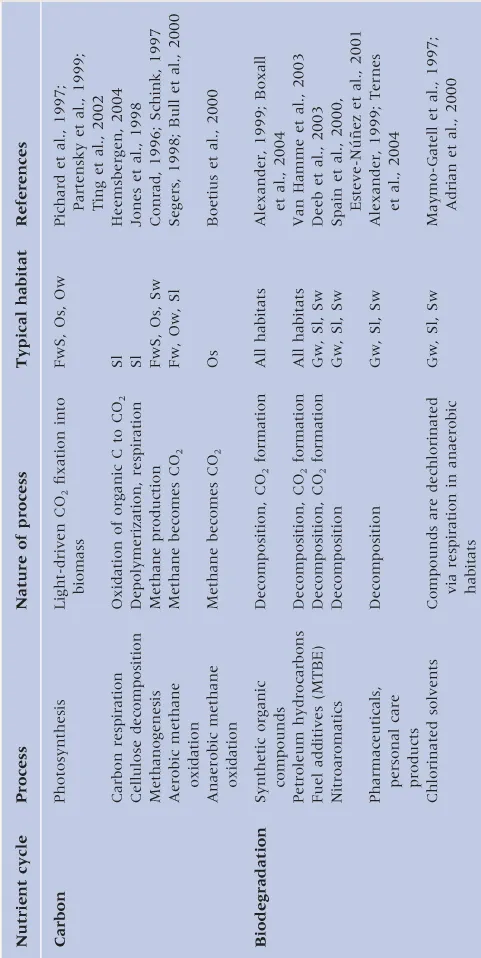
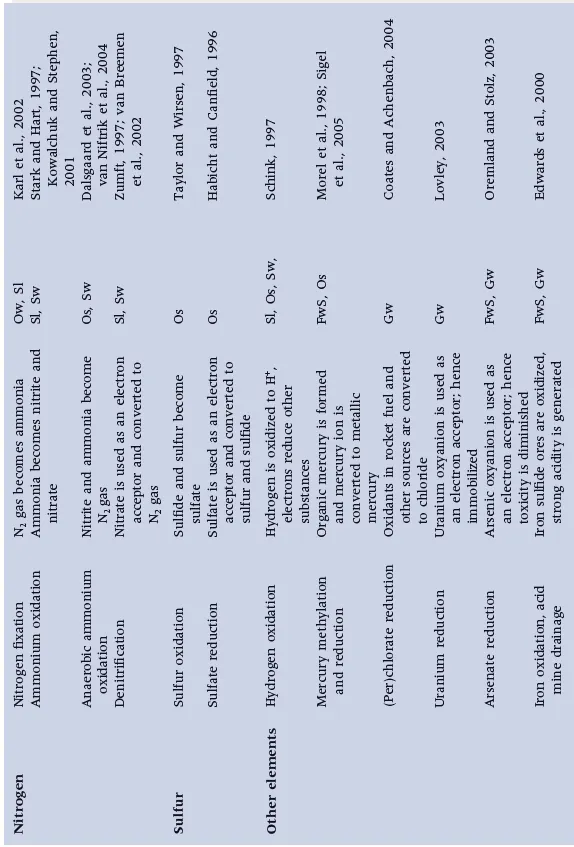
Fw, freshwater; FwS, freshwater sediment; Gw, groundwater; Os, ocean sediments; Ow, ocean waters; Si, soil; Sw, sewage.
1.3 A BRIEF HISTORY OF ENVIRONMENTAL MICROBIOLOGY
Early foundations of microbiology rest with microscopic observations of fungal sporulation (by Robert Hooke in 1665) and âwee animalculesâ - true bacterial structures (by Antonie van Leeuwenhoek in 1684). In the latter half of the nineteenth century, Ferdinand Cohn, Louis Pasteur, and Robert Koch were responsible for methodological innovations in aseptic technique and isolation of microorganisms (Madigan and Martinko, 2006). These, in turn, allowed major advances pertinent to spontaneous generation, disease causation, and germ theory.
Environmental microbiology also experienced major advancements in the nineteenth century; these extend through to the present. Environmental microbiology's roots span many continents and countries (Russia, Japan, Europe, and England) and a complex tapestry of contributions has developed. To a large degree, the challenges and discoveries in environmental microbiology have been habitat-specific. Thus, one approach for grasping the history and traditions of environmental microbiology is to recognize subdisciplines such as marine microbiology, soil microbiology, rumen microbiology, sediment microbiology, geomicrobiology, and subsurface microbiology. In addition, the contributions from various centers of training can also sometimes be easily discerned. These necessarily revolved around various investigators and the institutions where they were based.
As early as 1838 in Germany, C. G. Ehrenberg was developing theories about the influence of the bacterium, Gallionella ferruginea, on the generation of iron deposits in bogs (Ehrlich, 2002). Furthermore, early forays into marine microbiology by A. Certes (in 1882), H. L. Russell, P. Regnard, B. Fischer, and P. and G. C. Frankland allowed the completion of preliminary surveys of microorganisms from farranging oceanic waters and sediments (Litchfield, 1976).
At the University of Delft (the Netherlands) near the end of the nineteenth century, M. W. Beijerinck (Figure 1.1) founded the Delft School traditions of elective enrichment techniques (see Section 6.2) that allowed Beijerinck's crucial discoveries including microbiological transformations of nitrogen and carbon, and also other elements such as manganese (van Niel, 1967; Atlas and Bartha, 1998; Madigan and Martinko, 2006). The helm of the Delft School changed hands from Beijerinck to A. J. Kluyver, and the traditions have been continued in the Netherlands, Germany, and other parts of Europe through to the present. After training in Delft with Beijerinck and Kluyver, C. B. van Niel was asked by L. G. M. Baas Becking to established a research program at Stanford University's Hopkins Marine Station (done in 1929), where R. Y. Stainer, R. Hungate, M. Doudoroff and many others were trained, later establishing their own research programs at other institutions in the United States (van Niel, 1967).
S. Winogradsky (Figure 1.2) is regarded by many as the founder of soil microbiology (Atlas and Bartha, 1998). Working in the latter part of the nineteenth and early decades of the twentieth centuries, Winogradsky's career contributed immensely to our knowledge of soil and environmental microbiology, especially regarding microbial metabolism of sulfur, iron, nitrogen, and manganese. In 1949, much of Winogradsky's work was published as a major treatise entitled, Microbiologie du sol, problemes et methods: cinquante ans de recherches. Oeuvres Completes(Winogradsky, 1949).
Many of the marine microbiologists in the early twentieth century focused their attention on photoluminescent bacteria (E. Pluger, E. W. Harvey, H. Molisch, W. Beneche, G. H. Drew, and J. W. Hastings). Later, transformation by marine microorganisms of carbon and nitrogen were explored, as well as adaptation to low-temperature habitats (S. A. Waksman, C. E. ZoBell, S. J. Niskin, O. Holm-Hansen, and N. V. and V. S. Butkevich). The mid-twentieth century marine studies continued exploration of the physiological and structural responses of microorganisms to salt, low temperature, and pressure (J. M. Shewan, H. W. Jannasch, R. Y. Morita, R. R. Colwell, E. Wada, A. Hattori, and N. Taga). Also, studies of nutrient uptake (J. E. Hobbie) and food chains constituting the âmicrobial loopâ were conducted (L. R. Pomeroy).
Figure 1.1 Martinus Beijerinck (1851â1931). Founder of the Delft School of Microbiology, M. Beijerinck worked until the age of 70 at the University of Delft,the Netherlands. He made major discoveries in elective enrichment techniques and used them to advance theunderstanding of how microorganisms transform nitrogen, sulfur, and other elements. (Reproduced with permission from the American Society for Microbiology Archives, USA.)
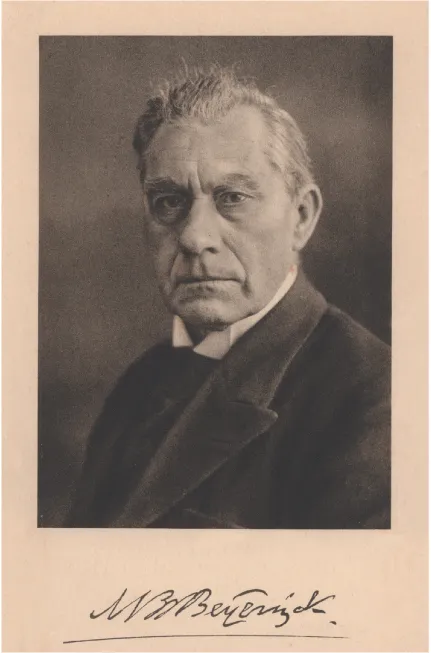
Figure 1.2 Sergei Winogradsky (1856â1953). A major contributor to knowledge of soil microbiology, S. Winogradsy described microbial cycling of sulfur and nitrogen compounds. He developed the âWinogradsky columnâ for growing diverse physiological types of aerobic and anaerobic, heterotrophic and photosynthetic bacteria across gradients of oxygen, sulfur, and light. (Reproduced with permission from the Smith College Archives, Smith College.)
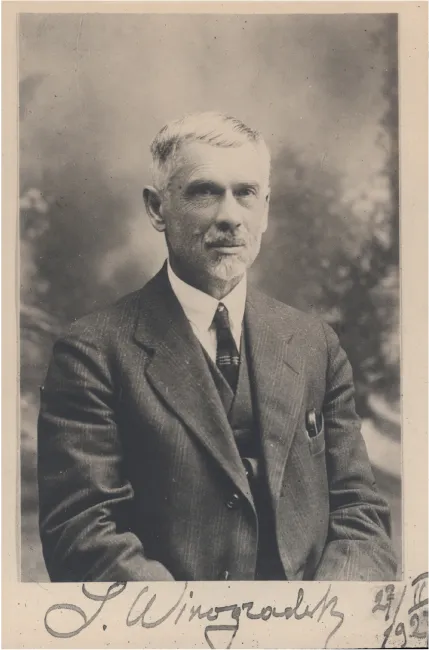
At Rutgers University, Selman A. Waksman was perhaps the foremost American scholar in the discipline of soil microbiology. Many of the Rutgers traditions in soil microbiology were initiated by J. Lipman,...