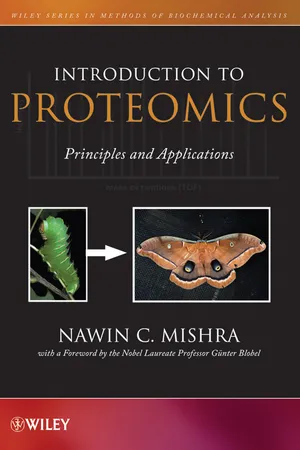
eBook - ePub
Introduction to Proteomics
Principles and Applications
Nawin C. Mishra
This is a test
Share book
- English
- ePUB (mobile friendly)
- Available on iOS & Android
eBook - ePub
Introduction to Proteomics
Principles and Applications
Nawin C. Mishra
Book details
Book preview
Table of contents
Citations
About This Book
Proteomics provides an introductory insight on proteomics, discussing the basic principles of the field, how to apply specific technologies and instrumentation, and example applications in human health and diseases. With helpful study questions, this textbook presents an easy to grasp and solid overview and understanding of the principles, guidelines, and especially the complex instrumentation operations in proteomics for new students and research scientists. Written by a leader in proteomics studies, Proteomics offers an expert perspective on the field and the future of proteomics.
Frequently asked questions
How do I cancel my subscription?
Can/how do I download books?
At the moment all of our mobile-responsive ePub books are available to download via the app. Most of our PDFs are also available to download and we're working on making the final remaining ones downloadable now. Learn more here.
What is the difference between the pricing plans?
Both plans give you full access to the library and all of Perlegoās features. The only differences are the price and subscription period: With the annual plan youāll save around 30% compared to 12 months on the monthly plan.
What is Perlego?
We are an online textbook subscription service, where you can get access to an entire online library for less than the price of a single book per month. With over 1 million books across 1000+ topics, weāve got you covered! Learn more here.
Do you support text-to-speech?
Look out for the read-aloud symbol on your next book to see if you can listen to it. The read-aloud tool reads text aloud for you, highlighting the text as it is being read. You can pause it, speed it up and slow it down. Learn more here.
Is Introduction to Proteomics an online PDF/ePUB?
Yes, you can access Introduction to Proteomics by Nawin C. Mishra in PDF and/or ePUB format, as well as other popular books in Biological Sciences & Biochemistry. We have over one million books available in our catalogue for you to explore.
Information
Chapter 1
Historical Perspectives
Biology becomes much more understandable in light of genetics (Ayala and Kiger 1984). This is true even more so in the case of the theory of evolution proposed by Darwin (1859). It seems the theory of evolution would have been placed on a solid foundation from the start if Darwin would have been aware of the Mendelian rules of inheritance. There is some indication that a copy of Mendel's publication was received by Darwin, which remained unopened during his lifetime. It is believed that this caused Darwin's failure to provide a firm basis on which selection works during the process of evolution.
Genetics has had several major breakthroughs during its development that have made biology a well-established discipline of science. Some of these break throughs are discussed here. The first major discovery was the rules of inheritance by Mendel (1866). This provided the particulate nature of inheritance and established the presence of genes, which control phenotypes. It also provided genes as the ultimate basis for propelling the process of evolution of organisms and integrated the different branches of the science of biology. In addition, Mendelian genetics transformed biology from a science based exclusively on observations to an experimental science where certain ideas could be tested by performing experiments.
The second major breakthrough was discovered by Beadle and Tatum (1941) with their conceptual one-geneāone-enzyme hypothesis. This proved the biochemical basis for the mechanism of gene action and integrated chemistry into biology. It provided the tool for analyzing metabolic pathways and several complex systems, including the nervous system. It also provided the understanding of the genetic basis of diseases and their possible cures by chemical manipulations and ultimately by gene therapy.
The discovery of the structure of DNA by Watson and Crick (1953) marked the third major breakthrough in biology. The discovery of the WatsonāCrick DNA structure was aptly meaningful in view of the findings of DNA as the chemical basis of inheritance (Avery et al. 1944, Hershey and Chase 1952). The WatsonāCrick structure of DNA provided the molecular basis for the understanding of the mechanisms of the storage and transmission of genetic information and possible changes (mutations) therein. Mutation provided the source of variations that could be selected for during the process of Darwinian evolution. Thus, the DNA structure created by Watson and Crick made genetics not only necessary but also unavoidable in the understanding of Darwin's evolution by natural selection. In 1962, Watson, Crick, and Wilkins received the Nobel Prize for this landmark discovery of the DNA structure.
The development of the WatsonāCrick structure of DNA led to the birth of molecular biology followed by the enunciation of the central dogma in biology. Molecular biology attempted to provide the molecular basis for everything in biology and biochemistry leading to the unity of life. Molecular biology perpetuated the reductionistic view of living systems: Reductionists attempt to understand a system by understanding its molecular components. Molecular biology also led to the development of a better understanding of diseases and their control by pharmaceuticals. The field of molecular biology ushered in by the WatsonāCrick DNA structure led to the development of scores of Nobel Prize-winning concepts in biology, biochemistry, and medicine as discussed later in this book.
The coming of genomics marked the fourth major breakthrough in biology. Advances in genome sequencing and availability of human and several other genome sequences by 2001 provided the basis for the understanding of the uniqueness of humans in possessing certain distinctive DNA segments. Genomics also provides the basis for the understanding of variations among individuals as differences in DNA sequences. Furthermore, it provides molecular insight into the genetic basis for differences in our response to the same drug. The variation in individual DNA sequences is expected to provide the molecular understanding of our several complex traits, including behavior. DNA sequences also provide a better insight into the record of the evolutionary processes in an organism. Genomics is expected to provide a better understanding of a complex organism like humans after the elucidation of the roles of noncoding sequences (introns) of DNA. Understanding the roles of introns is currently a formidable task: It is believed that the elucidation of the roles of introns will add a new dimension to the understanding of biology.
The fifth breakthrough underway is the development of proteomics. This is bringing a better understanding of biochemical pathways and the roles of protein interactions. Above all, proteomics provides a clue to answering the big question of how a small number of genes can control several phenotypes in a complex organism like humans. A major conceptual scheme emerging from proteomics is that it is the number of interactions of proteins and not the number of proteins per se that is responsible for the myriad phenotypes in an organism.
The sixth breakthrough that is in making involves the science of synthetic genetics which would allow creation of new organisms by creation of entirely new genomes or by the manipulation of existing ones with the help of the techniques of molecular genetics, genomics, proteomics and bioinformatics.
Advances in genomics and proteomics in conjunction with bioinformatics have made it possible to realize the dreams of the chemists of the 20th century. These chemists wanted to decipher the amino acid sequences of all proteins to understand their functions. Proteomics has made it possible to determine the amino acid sequence of any protein. In addition, future advances in genomics and proteomics are expected to bring several revolutions in medicine and will make personalized medicine a reality. Advances in proteomics are expected to integrate the reductionistic views of Watson and Crick into systems biology to show how molecular parts evolved and how they fit together to work as an organism. The latter is expected to provide the ultimate understanding of biology.
1.1 Introduction to Proteomics
The term āproteomeā originates from the words protein and genome. It represents the entire collection of proteins encoded by the genome in an organism. Proteomics, therefore, is defined as the total protein content of a cell or that of an organism. Proteomics is the understanding of the structure, function, and interactions of the entire protein content of an organism. Proteins control the phenotype of a cell by determining its structure and, above all, by carrying out all functions in a cell. Defective proteins are the major causes of diseases and thus serve as useful indicators for the diagnosis of a particular disease. In addition, proteins are the primary targets of most drugs and thus are the main basis for the development of new drugs. Therefore, the study of proteomics is important for understanding their role in the cause and control of diseases and in the development of humans as well as that of other organisms.
Proteins are encoded by DNA in most organisms and by RNA in some viruses. In all cases except RNA viruses, DNA is transcribed into RNA, which is then translated into a protein. In case of RNA virus, however, RNA is translated directly into proteins. Initially, it was thought that one gene makes one enzyme, which controls a phenotype. However, this view has undergone tremendous changes in the last several decades mainly because of the discovery of the split nature of eukaryotic genes, which involves RNA splicing, the occurrence of RNA editing, and the phenomenon of RNA silencing. The split nature of gene, RNA splicing, RNA editing, and RNA silencing are discussed later in this chapter.
In eukaryotes, the coding sequences of a gene called exons are interrupted by the noncoding stretches of nucleotides called introns. The exons are spliced after removal of introns within a gene continuously (referred to as cis splicing) or discontinuously (referred to as alternate splicing) or between exons of different genes leading to transsplicing. The different modes of splicing of exons and posttranslational modifications of proteins are responsible for the abundance of proteins in eukaryotic organisms. In humans there are approximately 23,000 genes and more than 500,000 proteins.
The findings of suppressor genes and the split nature of genes may present apparent contradictions to the one-geneāone-enzyme hypothesis. However, with the coming of central dogma (Crick, 1958, 1970, Watson 1965, Mattick 2003, Lewin 2004) in biology and elucidation of the genetic code (Leder and Nirenberg 1964, Khorana 1968), it is understandable how suppressor genes work. Thus, the mechanism of action of suppressor genes does not contradict the original ideas implicit in Beadle and Tatum's one-geneāone-enzyme concept to any extent as it appears superficially. In light of central dogma, it is understandable that certain genes or DNA segments may code for different proteins or that the coding section of protein in DNA is distributed across a huge expanse of DNA interrupted by the noncoding sequences. It has become obvious that the one-geneāone-enzyme concept applies only to genes that encode one polypeptide and not to genes that have a split nature and can code more than one protein. Thus, the one-geneāone-enzyme concept is limited to the nature of the gene itself, just as Mendelian rules of inheritance apply only to the genes located in the nucleus and not to the genes that are located elsewhere in the cell beyond the nucleus. Thus, the Mendelian inheritance pertains to the location of the genes, whereas the one-geneāone-enzyme concept is limited to the nature of the gene itself.
Obviously, what Beadle and Tatum suggested is not an axiom but a rule, and certain situations just represent exceptions to their profound rule. It seems that nature too has the British view of rule that āexceptions prove the rule.ā The history of science is full of such exceptions. The most glaring example of such an exception involves the central dogma in molecular biology described by Francis Crick, the codiscoverer of the DNA structure. Crick (1958, 1970) surmised that sequential information in DNA is transferred to RNA and then to protein from RNA and that the direction of this information transfer is fixed. However, later it was shown that RNA is reverse transcribed into DNA, and at times, messenger RNA (mRNA) is edited by the addition or removal of cytidine or uridine before its translation in to protein, which suggests that information in a DNA segment is not translated directly into protein as implicit in central dogma. This idea suggests that DNA makes RNA, which makes protein. Howard Temin and David Baltimore received the Nobel Prize in 1975 for demonstrating this reverse transfer of information from RNA to DNA. The other glaring example of such an exception includes the enzymes. It was James Sumner of the Cornell University who established that enzymes are proteins. Soon, enzymes became synonymous with proteins until Sydney Altman of Yale University and Thomas Cech of the University of Colorado showed independently that certain enzymes are made of RNA and not proteins. Sumner in 1946 and Altman and Cech in 1989 were awarded Nobel Prizes for their contributions to the science of chemistry. Thus, it seems that biology, like any other branch of science, is replete with instances of exceptions to the rules.
The Swedish scientist Berzelius (1838)1 named certain naturally occurring polymers as proteins. The fact that enzymes are proteins was established by Sumner (1946). Later, Sanger (1958)established that proteins are made up of a sequence of amino acids. The fact that an enzyme and a substrate (or an antibody and antigen) require precise complementary fit in their structures, just like a hand in a glove, to interact with each other was established by Linus Pauling in the 1940s. In addition to Sumner (1946), both Pauling (1954) and Sanger (1958) received Nobel Prizes for their work in chemistry. Most proteins have enzymatic functions, but several of them such as actin and fibrinoactin are structural components of cells. Proteins are major constituents of muscle, cartilage, and bones. Proteins are also responsible for the mobility of muscle cells. Certain proteins serve as receptors for different molecules or work as immunoglobulins or antigens, or proteins can serve as allergens or participate in transport of various molecules, such as oxygen or sex hormones. Many proteins are hormones, such as insulin or human growth hormone (HGH), which control important metabolic functions in humans and other organisms. The three-dimensional structure and chemical modifications of proteins are important for the understanding of their functions in different capacities.
Gorrod (1909) first described certain human disorders as inborn errors of metabolism and implied the genetic basis of these diseases. However, it was the genius of Beadle and Tatum (1941) that led to the establishment of the fact that a protein is encoded by a gene. Working with, Neurospora, they showed that the synthesis of a substance in a metabolic pathway was impaired in a mutant. They showed that by disabling the gene controlling the enzyme that catalyzed a biochemical reaction in a metabolic pathway, the mutant developed nutritional requirements for that substance. Such mutants could not be grown on a minimal medium, but their growth was possible only when a particular substance was added to the minimal medium. For example, a mutant with impaired synthesis of arginine could not be grown on a minimal medium, but its growth was possible only when arginine was added to the minimal medium. This method was also used to map the biochemical pathways.
Beadle and Tatum (1941) called this conceptual scheme the one-geneāone-enzyme hypothesis. This hypothesis has been modified in various ways. However, despite several exceptions to this rule of one gene encoding one enzyme, the main tenets of the one-geneāone-enzyme hypothesis have remained the cornerstone of biology. This concept has been instrumental for the merger of chemistry with genetics and for the development of molecular biology. This theory provides the standard method to assign a function to a protein by creating a mutant and then showing which protein has a defective function or which function has been impaired in a particular protein. Because of this hypothesis, it was possible to analyze and study viral, microbial, plant, and animal genetics. This has been the basis for creating knockout mutations and for in vitro mutagenesis. This hypothesis has proven crucial for the analysis of any basic genetic mechanism, such as DNA replication, repair, and recombination, and for establishing the role of a protein in any metabolic pathway. Finally, this theory by Beadle and Tatum has led to advances in agriculture, animal husbandry, pharmaceutical sciences, and medicine. The one-geneāone-enzyme hypothesis has been the basis for the understanding and alleviation of human diseases and for the development of gene therapy.
The one-geneāone-enzyme hypothesis implied that a mutant must have altered the protein. Beadle and Tatum could not demonstrate the defective nature of the protein in their mutants because of the lack of technology at that time. However, this was demonstrated first at the biochemical level by Mitchell and Lein (1948, Mitchell, et al. 1948) and by Yanofsky (1952, 2005a, 2005b) in tryptophan, which required mutants of Neurospora that lacked the enzyme tryptophan synthetase responsible for the synthesis of tryptophan. This concept was also demonstrated later at the molecular level by Ingram 1957 in the case of hemoglobin in persons who suffer from sickle cell anemia. Ingram showed that the sixth amino acid āglutamic acid,ā which is found in the hemoglobin of a normal person, is replaced by valine in the hemoglobin of a sickle cell person. This one change from glutamic acid to valine is the basis for the blood disorders in a sickle cell person. Later, many other mutants were shown to lack a protein altogether or possess proteins with altered amino acid(s).
The one-geneāone-enzyme theory also implied the correspondence in the ordered position of nucleotides in a gene with the position of amino acid in the protein encoded by that gene. This colinearity in the structure of a gene and that of a protein was demonstrated independently by Yanofsky et al. (1964) and by Sarabhai, et al. (1964), as discussed later in this chapter.
1.2 Proteome and Proteomics
1.2.1 Proteins as the Cell's Way of Accomplishing Specific Functions
The proteome is defined as the total proteins encoded by the genome of an organism. Proteomics is the science of describing the identification and features of the proteome of an organism.
The term āproteomeā was first used by Marc Wilkins in 1994 (Wilkins 1996). An effort to describe the total proteins of an ...