
eBook - ePub
The Outer Solar System
Jupiter, Saturn, Uranus, Neptune and the Dwarf Planets
Britannica Educational Publishing, Erik Gregersen
This is a test
- English
- ePUB (mobile friendly)
- Available on iOS & Android
eBook - ePub
The Outer Solar System
Jupiter, Saturn, Uranus, Neptune and the Dwarf Planets
Britannica Educational Publishing, Erik Gregersen
Book details
Book preview
Table of contents
Citations
About This Book
Beyond Earth's small, red neighbor Mars lie the gaseous, giant planets of the Outer Solar System. This book investigates these behemoths and dwarf planet Pluto, as well as other curiosities within the solar system's farthest reaches, such as asteroid fields and the Kuiper belt.
Frequently asked questions
How do I cancel my subscription?
Can/how do I download books?
At the moment all of our mobile-responsive ePub books are available to download via the app. Most of our PDFs are also available to download and we're working on making the final remaining ones downloadable now. Learn more here.
What is the difference between the pricing plans?
Both plans give you full access to the library and all of Perlegoâs features. The only differences are the price and subscription period: With the annual plan youâll save around 30% compared to 12 months on the monthly plan.
What is Perlego?
We are an online textbook subscription service, where you can get access to an entire online library for less than the price of a single book per month. With over 1 million books across 1000+ topics, weâve got you covered! Learn more here.
Do you support text-to-speech?
Look out for the read-aloud symbol on your next book to see if you can listen to it. The read-aloud tool reads text aloud for you, highlighting the text as it is being read. You can pause it, speed it up and slow it down. Learn more here.
Is The Outer Solar System an online PDF/ePUB?
Yes, you can access The Outer Solar System by Britannica Educational Publishing, Erik Gregersen in PDF and/or ePUB format, as well as other popular books in Physical Sciences & Astronomy & Astrophysics. We have over one million books available in our catalogue for you to explore.
Information
Topic
Physical SciencesSubtopic
Astronomy & AstrophysicsCHAPTER 1
ASTEROIDS
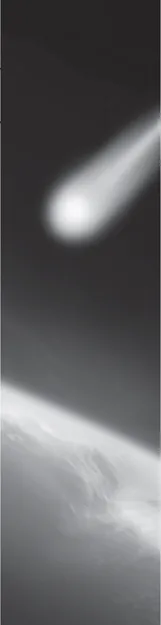
Beyond our planet, Earth, and the other terrestrial planetsâscorched Mercury, enshrouded Venus, and frigid Marsâlie the rocky bodies of the asteroid belt. Any natural solar system object other than the Sun, a planet, a dwarf planet, or a moon is called a small body; these include asteroids, meteoroids, and comets. Beyond those remnants of the early solar system lie the planets of the outer solar system. These planets, in order of their distance outward from the Sun, are Jupiter, Saturn, Uranus, and Neptune. Many astronomers consider the asteroid belt a demarcation point between the inner solar system (consisting mainly of the terrestrial planets) and the outer solar system.
Most of the rocky asteroids move around the Sun in elliptical orbits in the same direction of the Sunâs rotation. Such motion is termed prograde. Looking down on the solar system from a vantage point above Earthâs North Pole, an observer would find that prograde orbits are counterclockwise. Orbits in a clockwise direction are called retrograde.
Between the orbits of Mars and Jupiter are a host of rocky small bodies, about 1,000 km (600 miles) or less in diameter, called asteroids that orbit in the nearly flat ring called the asteroid belt. It is because of their small size and large numbers relative to the major planets that asteroids are also called minor planets. The two designations have been used interchangeably, though the term asteroid is more widely recognized by the general public. Among scientists, those who study individual objects with dynamically interesting orbits or groups of objects with similar orbital characteristics generally use the term minor planet, whereas those who study the physical properties of such objects usually refer to them as asteroids.
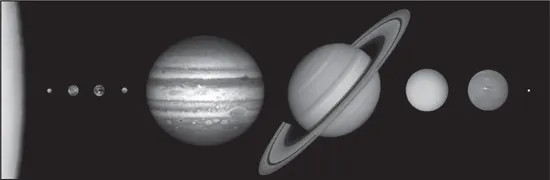
By comparison with those of the inner solar system, the planets of the outer solar system are veritable giants. Shown to scale, outward from the Sun, are Mercury, Venus, Earth, Mars, Jupiter, Saturn, Uranus, and Neptune. Also shown is the dwarf planet, Pluto. NASA/Lunar and Planetary Laboratory
MAJOR MILESTONES IN ASTEROID RESEARCH
The first asteroid was discovered on Jan. 1, 1801, by the astronomer Giuseppe Piazzi at Palermo, Italy. At first Piazzi thought that he had discovered a comet. However, after the orbital elements of the object had been computed, it became clear that the object moved in a planetlike orbit between the orbits of Mars and Jupiter. Owing to illness, Piazzi was able to observe the object only until February 11. As no one else was aware of its existence, it was not reobserved before it moved into the daytime sky. The short arc of observations did not allow computation of an orbit of sufficient accuracy to predict where the object would reappear when it moved back into the night sky, and so it was âlost.â
There matters might have stood were it not for astronomers searching for a âmissingâ planet between Mars and Jupiter during an astronomical conference in 1796. (Unfortunately, Piazzi was not a party to this attempt to locate the missing planet.) In 1801, German mathematician Carl Friedrich Gauss developed a method for computing the orbit of an asteroid from only a few observations. Using Gaussâs predictions, the German Hungarian astronomer Franz von Zach rediscovered Piazziâs âlostâ object on Jan. 1, 1802. Piazzi named this object Ceres after the ancient Roman grain goddess and patron goddess of Sicily, thereby initiating a tradition that continues to the present dayâasteroids are named by their discoverers.
The discovery of three more faint objects (at least when compared with Mars and Jupiter) in similar orbits over the next six yearsâPallas, Juno, and Vestaâcomplicated this elegant solution to the missing-planet problem and gave rise to the surprisingly long-lived though no longer accepted idea that the asteroids were remnants of a planet that had exploded. Following this flurry of activity, the search for the planet appears to have been abandoned until 1830, when Karl L. Hencke renewed it. In 1845 he discovered a fifth asteroid, which he named Astraea.
There were 88 known asteroids by 1866, when the next major discovery was made: Daniel Kirkwood, an American astronomer, noted that there were gaps (now known as Kirkwood gaps) in the distribution of asteroid distances from the Sun. The introduction of photography to the search for new asteroids in 1891, by which time 322 asteroids had been identified, accelerated the discovery rate. The asteroid designated (323) Brucia, detected in 1891, was the first to be discovered by means of photography. By the end of the 19th century, 464 had been found; this grew to more than 100,000 by the end of the 20th century and to more than four times that number by 2009. This explosive growth was a spin-off of a survey designed to find 90 percent of asteroids with diameters greater than 1 km (0.6 miles) that can cross Earthâs orbit and thus have the potential to collide with the planet..
LATER ADVANCES IN ASTEROID STUDIES
During much of the 19th century, most discoveries concerning asteroids were based on studies of their orbits. The vast majority of knowledge about the physical characteristics of asteroidsâfor example, their size, shape, rotation period, composition, mass, and densityâwas learned beginning in the 20th century, in particular since the 1970s. As a result of such studies, these objects went from being merely âminorâ planets to becoming small worlds in their own right.
In 1918 the Japanese astronomer Hirayama Kiyotsugu recognized clustering in three of the orbital elements (semimajor axis, eccentricity, and inclination) of various asteroids. He speculated that objects sharing these elements had been formed by explosions of larger parent asteroids, and he called such groups of asteroids âfamilies.â
In the mid-20th century, calculations of the lifetimes of asteroids whose orbits passed close to those of the major planets showed that most such asteroids were destined either to collide with a planet or to be ejected from the solar system on timescales of a few hundred thousand to a few million years. Since the age of the solar system is approximately 4.6 billion years, this meant that the asteroids seen today in such orbits must have entered them recently and implied that there was a source for these asteroids. At first this source was thought to be comets that had been captured by the planets and that had lost their volatile material through repeated passages inside the orbit of Mars. It is now known that most such objects come from regions in the main asteroid belt near Kirkwood gaps and other orbital resonances.
GEOGRAPHY OF THE ASTEROID BELT
Geography in its most literal sense is a description of the features on the surface of Earth or another planet. Three coordinatesâlatitude, longitude, and altitudeâsuffice for locating all such features. Similarly, the location of any object in the solar system can be specified by three parametersâheliocentric ecliptic longitude, heliocentric ecliptic latitude, and heliocentric distance. Such positions, however, are valid for only an instant of time since all objects in the solar system are continuously in motion. Thus, a better descriptor of the âlocationâ of a solar system object is the path, called the orbit, that it follows around the Sun or, in the case of a planetary satellite (moon), the path around its parent planet.
All asteroids orbit the Sun in elliptical orbits and move in the same direction as the major planets. Some elliptical orbits are very nearly circles, while others are highly elongated (eccentric). An orbit is completely described by six geometric parameters called its elements. Orbital elements, and hence the shape and orientation of the orbit, also vary with time because each object is gravitationally acting on, and being acted upon by, all other bodies in the solar system. In most cases, these gravitational effects can be accounted for so that accurate predictions of past and future locations can be made and a mean orbit can be defined. These mean orbits can then be used to describe the geography of the asteroid belt.
NAMES AND ORBITS OF ASTEROIDS
Because of their widespread occurrence, asteroids are assigned numbers as well as names. The numbers are assigned consecutively after accurate orbital elements have been determined. Ceres is officially known as (1) Ceres, Pallas as (2) Pallas, and so forth. Of the more than 450,000 asteroids discovered by 2009, about 47 percent were numbered. Asteroid discoverers have the right to choose names for their discoveries as soon as they are numbered. The names selected are submitted to the International Astronomical Union (IAU) for approval.
Prior to the mid-20th century, asteroids were sometimes assigned numbers before accurate orbital elements had been determined, and so some numbered asteroids could not later be located. These objects were referred to as âlostâ asteroids. The final lost numbered asteroid, (719) Albert, was recovered in 2000 after a lapse of 89 years. Many newly discovered asteroids still become âlostâ because of an insufficiently long span of observations, but no new asteroids are assigned numbers until their orbits are reliably known.
The Minor Planet Center at the Harvard-Smithsonian Center for Astrophysics in Cambridge, Mass., maintains computer files for all measurements of asteroid positions. As of 2009, there were more than 60 million such positions in its database.
DISTRIBUTION AND KIRKWOOD GAPS
The great majority of the known asteroids move in orbits between those of Mars and Jupiter. Most of these orbits, in turn, have semimajor axes, or mean distances from the Sun, between 2.06 and 3.28 AU, a region called the main belt. The mean distances are not uniformly distributed but exhibit population depletions, or âgaps.â These so-called Kirkwood gaps are due to mean-motion resonances with Jupiterâs orbital period. An asteroid with a mean distance from the Sun of 2.50 AU, for example, makes three circuits around the Sun in the time it takes Jupiter, which has a mean distance of 5.20 AU, to make one circuit. The asteroid is thus said to be in a three-to-one (written 3:1) resonance orbit with Jupiter. Consequently, once every three orbits, Jupiter and an asteroid in such an orbit would be in the same relative positions, and the asteroid would experience a gravitational force in a fixed direction. Repeated applications of this force would eventually change the mean distance of this asteroidâand others in similar orbitsâthus creating a gap at 2.50 AU. Major gaps occur at distances from the Sun that correspond to resonances with Jupiter of 4:1, 3:1, 5:2, 7:3, and 2:1, with the respective mean distances being 2.06, 2.50, 2.82, 2.96, and 3.28. The major gap at the 4:1 resonance defines the nearest extent of the main belt; the gap at the 2:1 resonance, the farthest extent.
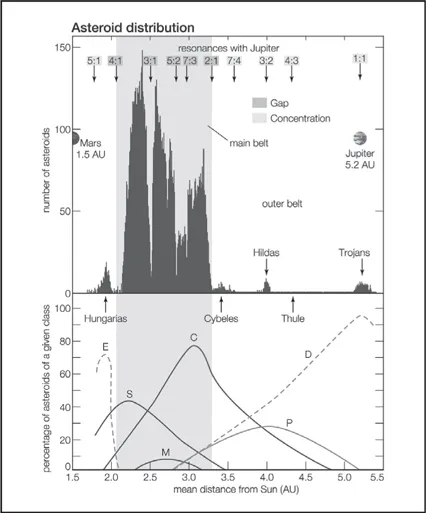
Asteroid distribution between Mars and Jupiter. (Top) Numbers of asteroids from a total of more than 69,500 with known orbits are plotted against their mean distances from the Sun. Major depletions, or gaps, of asteroids occur near the mean-motion resonances with Jupiter between 4:1 and 2:1, whereas asteroid concentrations are found near other resonances. The distribution does not indicate true relative numbers, because nearer and brighter asteroids are favoured for discovery. In reality, for any given size range, three to four times as many asteroids lie between the 3:1 and 2:1 resonances as between the 4:1 and 3:1 resonances. (Bottom) Relative percentages of six major asteroid classes are plotted against their mean distances. At a given mean distance, the percentages of the classes present total 100 percent. As the graph reveals, the distribution of the asteroid classes is highly structured, with the different classes forming overlapping rings around the Sun. EncyclopĂŚdia Britannica, Inc.
Some mean-motion resonances, rather than dispersing asteroids, are observed to collect them. Outside the limits of the main belt, asteroids cluster near resonances of 5:1 (at 1.78 AU, called the Hungaria group), 7:4 (at 3.58 AU, the Cybele group), 3:2 (at 3.97 AU, the Hilda group), 4:3 (at 4.29 AU, the lone asteroid (279) Thule), and 1:1 (at 5.20 AU, the Trojan groups). The presence of other resonances, called secular resonances, complicates the situation, particularly at the sunward edge of the belt. Secular resonances, in which two orbits interact through the motions of their ascending nodes, perihelia, or both, operate over timescales of millions of years to change the eccentricity and inclination of asteroids. Combinations of mean-motion and secular resonances can either result in long-term stabilization of asteroid orbits at certain mean-motion resonances, as is evidenced by the Hungaria, Cybele, Hilda, and Trojan asteroid groups, or cause the orbits to evolve away from the resonances, as is evidenced by the Kirkwood gaps.
NEAR-EARTH ASTEROIDS
Asteroids that can come close to Earth are called near-Earth asteroids (NEAs), although only some NEAs actually cross Earthâs orbit. NEAs are divided into several classes. Asteroids belonging to the class most distant from Earthâthose asteroids that can cross the orbit of Mars but that have perihelion distances greater than 1.3 AUâare dubbed Mars crossers. This class is further subdivided into two: shallow Mars crossers (perihelion distances no less than 1.58 AU but less than 1.67 AU) and deep Mars crossers (perihelion distances greater than 1.3 AU but less than 1.58 AU).
The next most distant class of NEAs is the Amors. Members of this group have perihelion distances that are greater than 1.017 AU, which is Earthâs aphelion distance, but no greater than 1.3 AU. Amor asteroids therefore do not at present cross Earthâs orbit. Because of strong gravitational perturbations produced by their close approaches to Earth, however, the orbital elements of all Earth-approaching asteroids except the shallow Mars crossers change appreciably on timescales as short as years or decades. For this reason, about half the known Amors, including (1221) Amor, the namesake of the group, are part-time Earth crossers. Only asteroids that cross the orbits of planetsâi.e., Earth-approaching asteroids and idiosyncratic objects such as (944) Hidalgo and Chironâsuffer significant changes in their orbital elements on timescales shorter than many millions of years.
There are two classes of NEAs that deeply cross Earthâs orbit on an almost continuous basis. The first of these to be discovered were the Apollo asteroids, named for (1862) Apollo, which was discovered in 1932 but was lost shortly thereafter and not rediscovered until 1978. The mean distances of Apollo asteroids from the Sun are greater than or equal to 1 AU, and their perihelion distances are less than or equal to Earthâs aphelion distance of 1.017 AU; thus, they cross Earthâs orbit when near the closest points to the Sun in their own orbits. The other class of Earth-crossing asteroids is named Atens for (2062) Aten, which was discovered in 1976. The Aten asteroids have mean distances from the Sun that are...
Table of contents
- Cover Page
- Title Page
- Copyright Page
- Contents
- Introduction
- Chapter 1: Asteroids
- Chapter 2: Meteors and Meteorites
- Chapter 3: Jupiter
- Chapter 4: Saturn
- Chapter 5: Uranus
- Chapter 6: Neptune
- Chapter 7: Pluto, the Kuiper Belt, and Beyond
- Chapter 8: Comets
- Appendix A: Moons of Jupiter
- Appendix B: Moons of Saturn
- Appendix C: Moons of Uranus
- Appendix D: Moons of Neptune
- Appendix E: Notable Kuiper Belt Objects
- Glossary
- For Further Reading
- Index