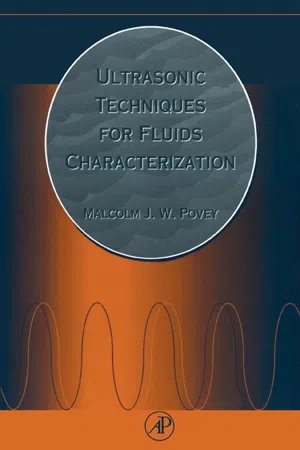
eBook - ePub
Ultrasonic Techniques for Fluids Characterization
Malcolm J.W. Povey
This is a test
- 214 pages
- English
- ePUB (mobile friendly)
- Available on iOS & Android
eBook - ePub
Ultrasonic Techniques for Fluids Characterization
Malcolm J.W. Povey
Book details
Book preview
Table of contents
Citations
About This Book
This book is a comprehensive and practical guide to the use of ultrasonic techniques for the characterization of fluids. Focusing on ultrasonic velocimetry, the author covers the basic topics and techniques necessaryfor successful ultrasound measurements on emulsions, dispersions, multiphase media, and viscoelastic/viscoplastic materials. Advanced techniques such as scattering, particle sizing, and automation are also presented. As a handbook for industrial and scientific use, Ultrasonic Techniques for Fluids Characterization is an indispensable guide to chemists and chemical engineers using ultrasound for research or process monitoring in the chemical, food processing, pharmaceutical, cosmetic, biotechnology, and fuels industries.
- Appeals to anyone using ultrasound to study fluids
- Provides the first detailed description of the ultrasound profiling technique for dispersions
- Describes new techniques for measuring phase transitions and nucleation, such as water/ice and oil/fat
- Presents the latest ultrasound techniques for particle sizing in concentrated systems
- Explains new techniques for compressibility measurements in dispersions and fluids, including cell suspensions
- Contains a detailed treatment of ultrasound scattering theory
- Written by one of the leading researchers in the field
- Includes over 350 references to the primary literature
Frequently asked questions
How do I cancel my subscription?
Can/how do I download books?
At the moment all of our mobile-responsive ePub books are available to download via the app. Most of our PDFs are also available to download and we're working on making the final remaining ones downloadable now. Learn more here.
What is the difference between the pricing plans?
Both plans give you full access to the library and all of Perlegoâs features. The only differences are the price and subscription period: With the annual plan youâll save around 30% compared to 12 months on the monthly plan.
What is Perlego?
We are an online textbook subscription service, where you can get access to an entire online library for less than the price of a single book per month. With over 1 million books across 1000+ topics, weâve got you covered! Learn more here.
Do you support text-to-speech?
Look out for the read-aloud symbol on your next book to see if you can listen to it. The read-aloud tool reads text aloud for you, highlighting the text as it is being read. You can pause it, speed it up and slow it down. Learn more here.
Is Ultrasonic Techniques for Fluids Characterization an online PDF/ePUB?
Yes, you can access Ultrasonic Techniques for Fluids Characterization by Malcolm J.W. Povey in PDF and/or ePUB format, as well as other popular books in Scienze fisiche & Fisica. We have over one million books available in our catalogue for you to explore.
Information
Topic
Scienze fisicheSubtopic
Fisica1
INTRODUCTION
1.1 THE BEGINNINGS
It is very easy to measure the velocity of sound. All you need is a stopwatch and a measuring tape. In 1827 Colladon and Sturm used a bell to produce a ring of sound in the waters of Lake Geneva. They used their ears as receiver and a stopwatch to time the clap of the bell after it traveled through the lake. They determined the distance between two boats, one on each side of the lake, with a tape measure and used the flash from a shot of gunpowder to signal the initial clap of the bell. In this way they found the velocity of sound in the waters of the lake. You can dispense with the colleague and the gunpowder if you can find a suitable canyon. Place yourself with your back to the canyon wall, then shout and start the stopwatch simultaneously. Stop the stopwatch when you hear your echo. Then measure the width of the canyon, double the distance, and divide it by the time on the stopwatch to obtain the velocity of sound.
The idea that sound is a wave phenomenon grew out of observations of water waves (Pierce, 1981a; Lindsay, 1973, in which many of the original papers referred to in subsequent paragraphs are reprinted). This idea is mentioned by the Greek philosopher Chrysippus (ca. 240 B.C.), by the Roman architect and engineer Vetruvius (ca. 25 B.C.), and by the Roman philosopher Boethius (a.d. 480â524). The wave idea was also promulgated by Aristotle (384â322 B.C.). However, the ancients did not have the technical apparatus necessary to exploit their ideas.
Newton (1642â1727) in his Principia (1686) first expounded the mechanical interpretation of sound as being âpressureâ pulses transmitted through neighboring fluid particles. The foundations for the present classical theory of sound propagation were laid by Euler (1707â1783), Lagrange (1736â1813), and dâAlembert (1717â1738), who gave continuum physics a definite mathematical structure. These developments coincided with the increasing availability of technical apparatus to permit their exploitationâespecially the clock, gun, and telescope (Hessen, 1931).
In the last century, sound velocity measurement was the standard method of determining the adiabatic compressibility of materials and hence the ratio of specific heats (Zemansky, 1957a); it was therefore a little-noticed but indispensable part of the science of thermodynamics. It has remained so to this day.
Today, sound velocity measurement is ubiquitous in industrial processes; go around any process plant and you are certain to find ultrasonic devices for level, concentration (see Chapter 2), and flow measurement (Sheppard, 1994) in liquids and gases. In metal fabrication, ultrasound nondestructive evaluation (NDE) and testing (NDT) are important quality control tools (Kuttruff, 1991b). Ultrasonic NDE is characterized today by frequencies between 0.1 and 50 MHz, pulsed operation and low power levels (<1 W mâ2). In civil engineering, ultrasound velocity measurement has its part to play in monitoring the porosity of concrete and the integrity of metal structures. The safety of ships depends on ultrasonic determination of the corrosion layer of shipsâ hulls.
Medical applications of ultrasound usually do not involve measurement of sound velocity, but instead depend on the relative invariance of sound velocity in human tissue. Many medical apparatuses measure the reflected signal and display the spatial variation of its amplitude, often using the time domain to give depth to the image (Kuttruff, 1991c). Blood flow is measured using ultrasound doppler (Fox, 1996).
The majority of the current industrial applications of ultrasound depend on the development from radar principles of the pulse-echo technique by Pellam and Galt (1946). Pellam and Gaitâs work forms the technical basis for most of the equipment described in this work.
The existing applications of ultrasound scrape the surface of what we can learn from this form of motion. To get a measure of the possibilities it is only necessary to look to nature. The bat actually âseesâ with ultrasound (Suga, 1990). With ultrasound echo ranging and backscatter, different species of bat can locate their quarry, identify and select the type of insect, and then catch it in the presence of obstacles, all at high velocity. With the metaphor of âcolorâ (relating to frequency variation) in mind, imagine what would be possible if we could âseeâ with ultrasound. Recent advances in technology make this objective a perfectly feasible one, and aspects of the technique are widely used in medical scanning and visualization.
1.2 UNDERSTANDING SOUND
Much of the theoretical basis for the analysis of sound velocity in liquids used here is found in Woodâs A Textbook of Sound, which was republished in 1964 (Wood, 1941a, 1964). Wood provides experimental and technical flesh for the bare bones of the theoretical work of Lord Rayleigh (Strutt, 1872, 1877, 1896) in the 19th century and Lamb in the early 20th century (Lamb, 1925), among many others. The theory of sound is firmly based in classical physics, in particular Newtonâs laws of motion, and most especially his second law, which relates force to acceleration through force = mass Ă acceleration. Newton developed a theory relating the velocity of sound to the compressibility and density. Laplace (1816) pointed out that the compressibility in this relationship was adiabatic, not isothermal as Newton thought. The theory of sound, as developed by Rayleigh, transcends the second law by its wavelike nature. It is interesting to note that Strutt (1872) discovered a famous result during his investigation of the scattering of sound. He showed that the sky is blue because of the fourth-power dependence on wavelength for scattering from small particles.
1.3 REPRESENTATIONS OF SOUND
As has already been stated, attempts to understand sound have drawn on analogies with water waves. Pictures and analogies are a very powerful method for understanding sound. Wave representations of sound give a picture of the collective motion of large numbers of particles, whose individual motions still need to be recognized (Pierce, 1981a). This work is mainly concerned with a special case of sound propagation in which the particle motions which constitute the pressure pulses are parallel to the direction of propagation of the pulse. This is called compression or longitudinal sound. Unless stated otherwise, we shall always mean this type of sound wave. A representation of compression sound is given in Figure 1.1.
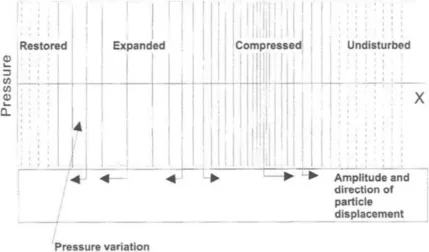
FIGURE 1.1 Particle position, particle displacement, and spatial pressure variation plotted against position (x) for a single cycle, sinusoidal, plane-traveling wave in a fluid medium. (Adapted from Pierce, 1981a.)
In contrast, the shear mode of propagation of sound is supported over macroscopic distances in solids but not in liquids. In the shear mode, particle displacement is transverse to the direction of propagation (Figure 1.2). There are other possible modes of acoustic propagation, but these are of little relevance to this work.
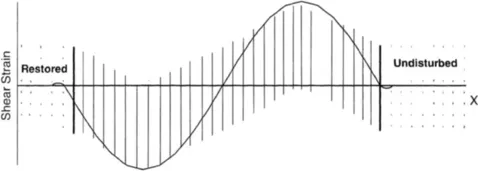
FIGURE 1.2 Particle position, particle displacement, and spatial shear strain variation plotted against position (x) for a single cycle, sinusoidal, plane-traveling shear wave.
In nearly every application of ultrasound to be discussed here, a special type of ultrasound source, called the piston source, is used. Where wave phenomena are concerned, the nature of the source and detector cannot be separated from the nature of the interaction of sound with the material under investigation. The performance of the transducer depends on its acoustical matching to the medium under investigation, as well as its electromagnetic matching to the detection and generation circuitry. The sound field in front of a piston source (Figure 1.3) may be represented using an analogy with water waves first employed by Newton to describe sound. In this representation, lines are drawn which coincide with the crests and troughs of the wave (this method of representing sound waves is used in Figure 1.1 as well). These lines are also lines of constant âphase.â The phase of a wave refers to the position of maximum or minimum in a wave (the peaks and troughs just referred to), relative to the waveform as a whole.
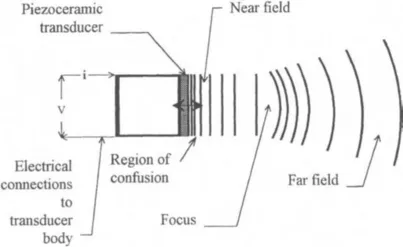
FIGURE 1.3 Piston source operating with the diameter (aperture) very much greater than the acoustic wavelength in the medium in contact with the front of the transducer.
It can be seen from Figure 1.3 that the sound field in front of the piston source is a very complicated one. The implications of this will ...
Table of contents
Citation styles for Ultrasonic Techniques for Fluids Characterization
APA 6 Citation
Povey, M. (1997). Ultrasonic Techniques for Fluids Characterization ([edition unavailable]). Elsevier Science. Retrieved from https://www.perlego.com/book/1835841/ultrasonic-techniques-for-fluids-characterization-pdf (Original work published 1997)
Chicago Citation
Povey, Malcolm. (1997) 1997. Ultrasonic Techniques for Fluids Characterization. [Edition unavailable]. Elsevier Science. https://www.perlego.com/book/1835841/ultrasonic-techniques-for-fluids-characterization-pdf.
Harvard Citation
Povey, M. (1997) Ultrasonic Techniques for Fluids Characterization. [edition unavailable]. Elsevier Science. Available at: https://www.perlego.com/book/1835841/ultrasonic-techniques-for-fluids-characterization-pdf (Accessed: 15 October 2022).
MLA 7 Citation
Povey, Malcolm. Ultrasonic Techniques for Fluids Characterization. [edition unavailable]. Elsevier Science, 1997. Web. 15 Oct. 2022.