
eBook - ePub
Foundations of Cognitive Processes
Robert W. Thatcher, E. Roy John
This is a test
Share book
- 382 pages
- English
- ePUB (mobile friendly)
- Available on iOS & Android
eBook - ePub
Foundations of Cognitive Processes
Robert W. Thatcher, E. Roy John
Book details
Book preview
Table of contents
Citations
About This Book
Originally published in 1977, this title describes the basic structure and function of the brain, as well as the highest cognitive functions, using data from various disciplines to detail ways in which behaviorally relevant functions are mediated by the neural systems. Among the topics discussed are the neurophysiology of emotion, the chemical basis of memory, daily subjective experience and psychopathology, and information representation. A major purpose of this volume was to provide the student not only with a sound foundation in functional neuroscience, but also to equip them with a detailed understanding of how these facts and methods can be applied to clinical problems.
Frequently asked questions
How do I cancel my subscription?
Can/how do I download books?
At the moment all of our mobile-responsive ePub books are available to download via the app. Most of our PDFs are also available to download and we're working on making the final remaining ones downloadable now. Learn more here.
What is the difference between the pricing plans?
Both plans give you full access to the library and all of Perlegoâs features. The only differences are the price and subscription period: With the annual plan youâll save around 30% compared to 12 months on the monthly plan.
What is Perlego?
We are an online textbook subscription service, where you can get access to an entire online library for less than the price of a single book per month. With over 1 million books across 1000+ topics, weâve got you covered! Learn more here.
Do you support text-to-speech?
Look out for the read-aloud symbol on your next book to see if you can listen to it. The read-aloud tool reads text aloud for you, highlighting the text as it is being read. You can pause it, speed it up and slow it down. Learn more here.
Is Foundations of Cognitive Processes an online PDF/ePUB?
Yes, you can access Foundations of Cognitive Processes by Robert W. Thatcher, E. Roy John in PDF and/or ePUB format, as well as other popular books in Psychologie & Neurosciences cognitives et neuropsychologie. We have over one million books available in our catalogue for you to explore.
Information
1
Basic Neurophysiology
I. Fundamentals of Neurophysiology
This chapter is intended to provide a brief outline of the fundamentals of neurophysiology. More detailed information on this subject can be found in the following references: Brazier (1958); Ochs (1965); Purpura (1959); Quarton, Melnechuk, and Schmitt (1967); and Ruch, Patton, Woodbury, and Towe (1965).
A. Basic Structure
The brain is constituted of three basic compartments: glial cells, the extracellular space, and nerve cells or neurons. Glial cells, consisting primarily of astroglia and Oligodendroglia, form a close and interactive relationship with neurons. They provide metabolic and structural support for the nerve cells. They also appear to serve a reciprocal, âsymbioticâ function by exchanging proteins with neurons (HydĂ©n, 1967) and by regulating ionic concentrations in both the intracellular and extracellular space (Karahashi & Goldring, 1966; Nicholls & Kuffler, 1964). The extracellular space and glial cells together provide the structural and functional matrix in which the neurons are embedded.
The extracellular space (sometimes referred to as the intercellular space) is the smallest (15 to 20%) of the compartments. However, through this space flow essential metabolic substances and ionic materials related to the polarization and depolarization of neuronal membranes and related as well to the relatively powerful currents generated by the activity of synchronized masses of neurons. The term âextracellular spaceâ is misleading. Actually, this space is occupied by an extracellular structure. Electronmicrographs reveal an extracellular âfuzzâ made of branching mucopolysaccharides and glycosaccharides extending from a protein backbone on the outer surface of neuronal membranes (Fig. 1.1). The complexly branching saccharides contain positively and negatively charged sites that bind extracellular ions. Weak electrical currents, such as those used in measurements of tissue impedance, pass through the extracellular space with very little, if any, penetration of the membranes of neurons or glia (Cole, 1940). Extracellular fluids have a specific resistance of approximately 4 Ω cmâ2 as compared with resistances of neurons or neuroglia which are approximately 5000 Ω cmâ2 (Coombs, Curtis, & Eccles, 1959; Nicholls & Kuffler, 1964).
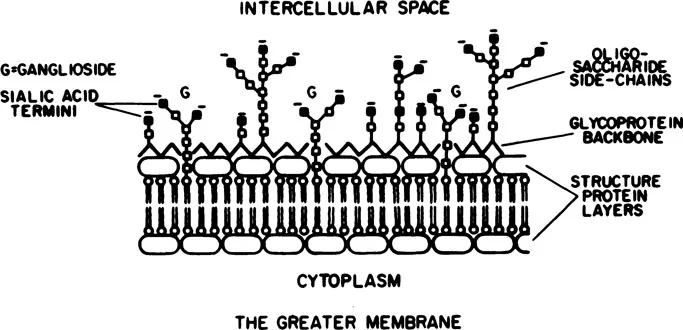
FIG. 1.1 Model of the neuronal membrane illustrating the glycoprotein and polysaccharides that form the extracellular âfuzz.â (From Lehninger, 1968.)
Impedance measurements which reflect conductance within the extracellular channels demonstrate changes during learning, suggesting that structural alterations of the extracellular matrix underlie information storage and retrieval (Adey, Kado, & Didio, 1962; Adey, Kado, Didio, & Schindler, 1963; Adey, Kado, Mcllwain & Walter, 1966b). The greatest concentration of extracellular mucopolysaccharides is around synaptic junctions, the axon hillock, and axonal nodes, three regions which are fundamental in the generation of electrical impulses in neurons (Meyer, 1969). Conformational changes in the extracellular matrix may modify neural excitability by affecting ionic binding affinities at these critical sites. As will be emphasized in later sections, the electroencephalogram (EEG) involves complex current flows within small channels that branch profusely throughout this extracellular matrix.
The third compartment is comprised of neurons. The neuron consists of three functional portions: (a) a cell body or soma which contains genetic material and protein synthesizing mechanisms. The soma also contains specialized receptive membrane structures (at synapses) that allow the neuron to be influenced directly by other neurons. Extending from the soma are (b) dendrites which can be considered as receptive surfaces and (c) an axon which transmits impulses (Fig. 1.2). An axon is usually several times longer than its diameter and in the peripheral nervous system can be as great as one meter in length.
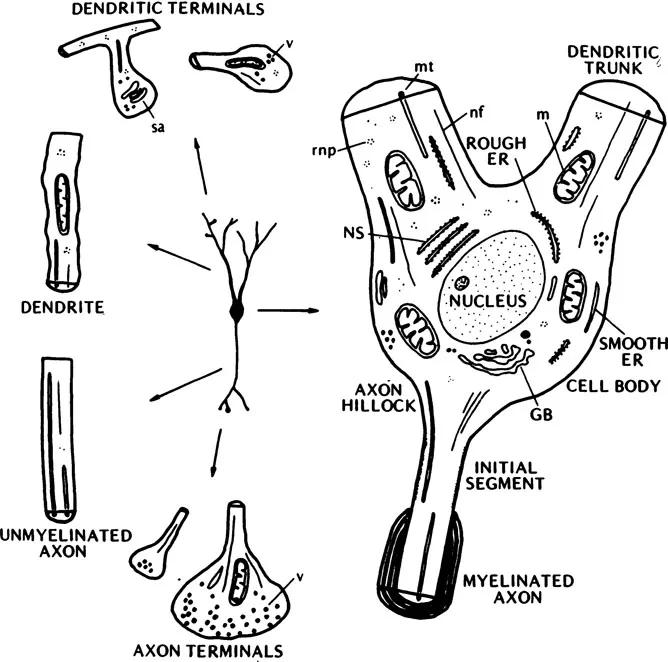
FIG. 1.2 An illustration of a neuron showing the cell body, axon, and dendrites as well as the interneuronal structure. ER, endoplasmic reticulum; GB, Golgi body; NS, Nissl substance; mt, microtubule; nf, neurofilament; rnp, ribonucleic particles; sa, spine apparatus; v, vesicles; m, mitochondria. (From Shepherd, 1974.)
Neurons are electrically excitable. Slow electrical potentials generated in the receptive regions (called synaptic potentials) give rise to impulses (called spikes or action potentials) that are transmitted down the axon. The axon arises from the soma at a specialized region called the axon hillock.
In an electronmicroscope, the soma can be seen to be limited by a double membrane structure which encloses a number of subcellular constituents: endoplasmic reticulum and ribosomes, nucleus and nucleolus, mitochondria, golgi apparati, and neurotubules. The neurotubules form a complex network serving the function of transporting cellular constituents to dendrites and axon terminals. Recent evidence shows that cell bodies strategically located in the brain stem (nucleus coeruleus, nucleus raphe, nucleus substantia nigra) give rise to axons that travel great distances to synapse with the limbic system, the cortex, and the extrapyramidal motor system. Through these axons flow chemical transmitters (norepinephrine, serotonin, dopamine) which play important roles in the regulation of emotion, arousal, and motor-sensory coordination. These transmitters are probably transported through the neurotubules. The function of these chemicals will be discussed in Section III.
The importance of neurotubules has been further emphasized by the discovery that aging is accompanied by a flattening of the dendritic neurotubules (Wis-niewski, Coblentz, & Terry, 1972). Malfunction of the transport mechanisms appears to be responsible, in part, for diminished function of brain cells in the very old.
In addition to the double membrane structure seen in the soma, long axons are enclosed by an insulating cover (myelin sheath) which functions to enable rapid and constant transmission velocities. The myelin sheath is in reality two membranes formed by specialized glial cells (Schwann cells) that wrap around the axon many times to form a multilayered structure (Fig. 1.3). The myelin is interrupted at regular intervals of approximately 0.5 to 1.5 mm along the fiber, by Nodes of Ranvier. The distance between the nodes, the internodal length, vary with axon diameter, age, and species (Hiscoe, 1947; Tasaki, 1953). The myelin sheath is a prominent feature of the long-axoned neurons; that is, those which form the peripheral sensory and motor systems and the long association bundles within the central nervous system (CNS). In the shortest axoned cells only one layer of myelin is present. As a consequence, a myelin sheath is not evident in the electronmicroscope. Over 97% of the cells comprising the CNS (called interneurons or association neurons) have very short axons without myelin sheaths.
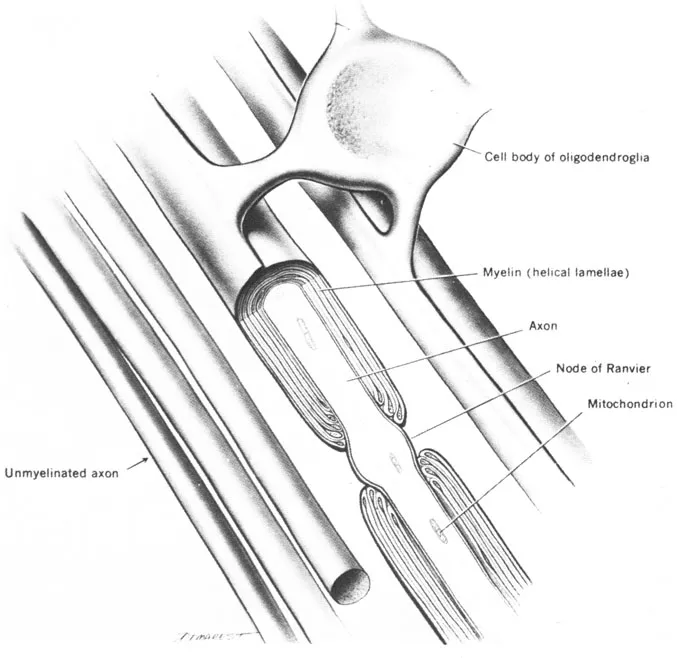
FIG. 1.3 The formation of myelin by Oligodendroglia. (From The Human Nervous System by C. R. Noback & R. J. Demarest. Copyright © 1975 by McGraw-Hill Book Company. Used with permission of McGraw-Hill Book Company.)
B. Action Potential
In the resting state, the inside of the axon is approximately 90 mV more negative than the outside. This potential difference, sometimes referred to as the transmembrane potential or simply the resting membrane potential, is caused by an imbalance in the concentration of ions (particularly sodium and potassium) across the membrane. Figure 1.4 illustrates the various ionic constituents that give rise to the potential difference. The concentration gradients are created by an active metabolic pump mechanism. When a metabolic poison such as Ouabain is administered, the gradients disappear.
There are two forces that act to alter the distribution of the ions across the membrane. One is caused by the individual concentration gradients for each ion, which tend to drive sodium into the cell and potassium and chloride out of the cell. The second is caused by the electromotive force produced by the imbalance of total ions across the membrane, which tends to drive potassium and sodium into the cell and chloride out of the cell. A succinct description of these interactive forces is given by the Nernst equation which expresses the equilibrium potential, that is, the condition where the potential difference across the membrane for a given ion is zero. The Nernst equation is
where V is the equilibrium potential for the ion, R is the gas constant, T is the absolute temperature, Z is the ionic valence, F is the Faraday (which equals 96,500 coulombs), and the quantities in parentheses are the concentration of the ion inside the membrane, (C)i, and outside the membrane, (C)o.
Due to concentration differences, the tendency for CI- ions and K+ ions to diffuse out of the cell is almost counterbalanced by the resting membrane potential. This balanced situation is not present in the case of Na+. Both the concentration gradient and the electrochemical force tend to drive Na+ into the cell.
The resting equilibrium potentials expressed by the Nernst equation are ~ -97 mV for K+ and ~ - 90 mV for CI-. The electromotive force necessary to achieve equilibrium for a resting membrane potential of-90 mV, is -7 mV for K+ and 0 m...