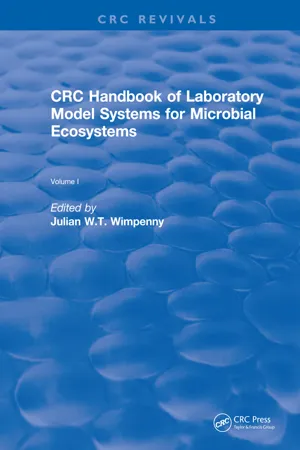
eBook - ePub
Revival: CRC Handbook of Laboratory Model Systems for Microbial Ecosystems, Volume I (1988)
Julian W.T. Wimpenny
This is a test
- 268 Seiten
- English
- ePUB (handyfreundlich)
- Über iOS und Android verfügbar
eBook - ePub
Revival: CRC Handbook of Laboratory Model Systems for Microbial Ecosystems, Volume I (1988)
Julian W.T. Wimpenny
Angaben zum Buch
Buchvorschau
Inhaltsverzeichnis
Quellenangaben
Über dieses Buch
These volumes present the main classes of useful laboratory model systems used to study microbial ecosystems, with emphasis on the practical details for the use of each model. The most commonly used model, the homogeneous fermenter, is featured along with linked homogeneous culture systems, film fermenters, and percolating columns. Additionally, gel-stabilized culture systems which incorporate molecular diffusion as their main solute transfer mechanism and the microbial colony are explained. Chapters comparing model systems with "microcosms" are included, along with discussions of the value of computer models in microbial ecosystem research. Highlighted is a global discussion of the value of laboratory models in microbial ecology.
Häufig gestellte Fragen
Wie kann ich mein Abo kündigen?
Gehe einfach zum Kontobereich in den Einstellungen und klicke auf „Abo kündigen“ – ganz einfach. Nachdem du gekündigt hast, bleibt deine Mitgliedschaft für den verbleibenden Abozeitraum, den du bereits bezahlt hast, aktiv. Mehr Informationen hier.
(Wie) Kann ich Bücher herunterladen?
Derzeit stehen all unsere auf Mobilgeräte reagierenden ePub-Bücher zum Download über die App zur Verfügung. Die meisten unserer PDFs stehen ebenfalls zum Download bereit; wir arbeiten daran, auch die übrigen PDFs zum Download anzubieten, bei denen dies aktuell noch nicht möglich ist. Weitere Informationen hier.
Welcher Unterschied besteht bei den Preisen zwischen den Aboplänen?
Mit beiden Aboplänen erhältst du vollen Zugang zur Bibliothek und allen Funktionen von Perlego. Die einzigen Unterschiede bestehen im Preis und dem Abozeitraum: Mit dem Jahresabo sparst du auf 12 Monate gerechnet im Vergleich zum Monatsabo rund 30 %.
Was ist Perlego?
Wir sind ein Online-Abodienst für Lehrbücher, bei dem du für weniger als den Preis eines einzelnen Buches pro Monat Zugang zu einer ganzen Online-Bibliothek erhältst. Mit über 1 Million Büchern zu über 1.000 verschiedenen Themen haben wir bestimmt alles, was du brauchst! Weitere Informationen hier.
Unterstützt Perlego Text-zu-Sprache?
Achte auf das Symbol zum Vorlesen in deinem nächsten Buch, um zu sehen, ob du es dir auch anhören kannst. Bei diesem Tool wird dir Text laut vorgelesen, wobei der Text beim Vorlesen auch grafisch hervorgehoben wird. Du kannst das Vorlesen jederzeit anhalten, beschleunigen und verlangsamen. Weitere Informationen hier.
Ist Revival: CRC Handbook of Laboratory Model Systems for Microbial Ecosystems, Volume I (1988) als Online-PDF/ePub verfügbar?
Ja, du hast Zugang zu Revival: CRC Handbook of Laboratory Model Systems for Microbial Ecosystems, Volume I (1988) von Julian W.T. Wimpenny im PDF- und/oder ePub-Format sowie zu anderen beliebten Büchern aus Medicine & Medical Theory, Practice & Reference. Aus unserem Katalog stehen dir über 1 Million Bücher zur Verfügung.
Information
Chapter 1
INTRODUCTION
This book is devoted to a description of some of the laboratory model systems which can usefully be applied to investigations of microbial growth and interactions where they are most at home, in their natural habitats.
It is accepted from the start that natural ecosystems are nearly always extraordinarily complex genotypically, structurally, and dynamically. It is for this reason that I and all the contributors to this volume advocate the judicious use of model systems, which, if chosen wisely and if their limitations are constantly born in mind, can throw light on the operation of microbes in the natural world.
MODELS AND MICROCOSMS
First we must discuss terminology. The terms “microcosm” and “model system” are sometimes used incautiously to signify the same thing. It is clear however that the two are conceptually quite different. Parkes1 suggests that the term microcosm defines “… a laboratory system which attempts to actually simulate as far as possible the conditions prevailing in the environment or part of the environment under study”. As is pointed out by Burns (Volume II, Chapter 3), the term microcosm can be totally misinterpreted to mean “site of microbial activity”. There may, however, be a consensus among other microbiologists, in which a microcosm possesses some or all of the following properties:
1.Origin. Microcosms derive from natural ecosystems.
2.Isolation. The microcosm, whatever its origin, is physically enclosed and no longer in contact with the natural ecosystems.
3.Size. Though variable in size, microcosms tend to be compact subsets of the natural system from which they came.
4.Genotypic heterogeneity. With some exceptions, most microcosm work uses natural mixed cultures of microorganisms.
5.Spatial heterogeneity. Although spatial heterogeneity is not in any way implied by the term microcosm, virtually all of the latter retain this property to some degree.
6.Temporal heterogeneity. The majority of microcosms are closed or partially closed systems, where time-dependent changes in the physical, chemical, and biological properties of the system are seen.
At first sight, a microcosm seems just to be a convenience, a piece of natural ecology tamed and brought into the laboratory without changing anything within it.
The main point in favor of the microcosm is that environmental factors (light, temperature, chemistry, etc.) can be manipulated, prolonging, for example, certain seasons or simplifying systems, perhaps eliminating diurnal variations.
It should be emphasized that however good the intention is to accurately reproduce the natural ecosystem, the microcosm is almost certain to suffer as a result of scale changes. As an “island” community isolated from the original system, there may be successional changes in species composition and concommitant changes in physical chemistry aggravated by the presence of the container boundary. For instance, if the latter is glass and the system is illuminated, photosynthetic species will proliferate. A good example of such a microcosm is the Winogradsky column2 which is derived from a sediment ecosystem. Sediment and water are placed over a source of carbon (usually cellulose) and a mixture of calcium sulfate and calcium carbonate in a glass vessel. If the container is kept in normal daylight, colored photosynthetic species develop as a series of pigmented bands. Clearly they mark vertical heterogeneity. On the other hand, by their presence and by the input of light energy to these zones, they contribute another level of heterogeneity at right angles to the vertical stratification already present in the sediment. This secondary heterogeneity is of course absent from the natural sediment, since the latter is never illuminated. These wall effects plus the addition of cellulose, gypsum, and chalk to the system mean that the Winogradsky column is no longer homologous with the sediment from which it originated. So is it microcosm or model? It is apparent that the Parkes definition cannot help here. The Winogradsky column obviously does not aim to simulate as exactly as possible the natural sediment. It does start from the natural system but equally it manipulates it. Perhaps a better definition which embraces these feelings is
A microcosm is a laboratory subset of the natural system from which it originates but from which it also evolves.
Such a brief definition suggests that if we establish a sample of a natural microbial ecosystem in the laboratory, it should still be termed a microcosm even if it is altered spatially or in terms of its physical chemistry. Microcosms allow a systematic examination of the responses of natural communities to environmental manipulation.
The model, on the other hand, is always an abstraction. The model never aims to reproduce the entire system in the laboratory. It always seeks to examine the properties of a part of the system ignoring or holding constant all the remaining factors. There may be any number of models investigating particular properties of the system. Some of these may be extremely simple. To grow a pure culture of a sulfate-reducing bacterium in a chemostat is to create a restricted model of one component of a sedimentary ecosystem. Such a model is perfectly valid if its limitations (spatial and temporal homogeneity, the absence of other species, the absence of different phases, and so on) are accepted.
Another model of a sedimentary system might recognize the importance of molecular diffusion and species diversity. The sulfate reducer could be incorporated into a gel stabilized system together with a sulfide oxidizing species. The two operating together in counter gradients of oxygen and a carbon source could carry out the chemical reactions of the sulfur cycle found in the upper levels of natural sediment ecosystems.
The model could be made even more sophisticated. Specific mineral particles and a greater range of substrates and species could be incorporated into the gel. The model system becomes closer to the natural ecosystem with each level of sophistication. At some point of course one could conceivably say “the model is the system …!” This is probably not the main virtue of models, however. The modeler may well consider that to travel hopefully is better than to arrive. To understand completely a particular ecosystem is probably less important than to discern rules of behavior which apply at a fundamental level to many different ecosystems. The model, if designed well, can by virtue of abstraction and simplification offer this possibility.
The difference between model and microcosm can, for some people, epitomize the difference between an essentially reductionist approach to understanding ecology on the one hand, and a holistic approach on the other. The reductionist believes that understanding the simplified subunits allows one to predict the properties of system from which they derived. Thus, if the properties of pure cultures of bacteria are totally understood, then their behavior in a natural community and hence the behavior of the community itself can be confidently predicted. The holist, on the other hand, believes that breaking a system down into component parts loses part of its veracity. A holist philosophy considers that the whole is greater than the sum of its component parts.
It is likely that both approaches are valid, essential even, to the prosecution of experimental science. Clearly, higher levels of complexity bring the need for new rules, new properties, new types of order. After all, a complete understanding of the properties of bricks does not help to comprehend the rules of design or architecture needed to construct a Victorian railway station! On the other hand, systems can be too complicated to comprehend as they stand. It must then be right to take a reductionist stance, at least at first, if this gives a clear understanding of some part of the natural system. If the model is very simple, the information too will be simple. Perhaps if we understand some of the properties of bricks, for example their mechanical strength, we can at least predict how tall we can build our railway station before it collapses. In the same way, understanding the biochemical behavior of a single species can give some insight into the way in which it could behave in a complex community. Once the simple model has been deployed and all the relevant information collated, it is time to sophisticate the model. The next level of complexity can now be investigated and comprehended. This is not really a reductionist approach at all. Simplification merely establishes a platform upon which higher levels of sophistication can rest more securely.
Ought reductionism be distinguished from the normal scientific processes of analysis and synthesis? The reductionist studies elements of a system and is then content to predict the behavior of the system as a whole. The “normal” scientist carefully dissects the whole system before reassembling increasingly more complex subsets of it. Pursued to its logical conclusion, even this distinction fades and reductionism is seen as just one extreme of the normal processes of analysis and synthesis.
In the meantime, right at the other end of this scale, the holist is investigating the system just as it stands. Some of his findings must inevitably be descriptive and may not be easy to interpret at the most basic level; however the holist performs the vital function of searching for and documenting the emergent properties of the complete system. It seems clear that holism and the sort of “reductionism” described here move together and at some point lead to a unified interpretation of the complete system which will satisfy all sides.
Holism and reductionism are not in the end very useful terms, since they simply represent two extreme attitudes to science. The advocate of one believes in starting simple and only increasing the complexity of a problem when it is necessary to do this, while the advocate of the other can only comprehend the entire system and is motivated to study this from an aesthetic sense of unity and even beauty that the system as a whole displays. Finally, science as a process is flexible enough to embrace all sorts of approaches and can accomodate, and indeed gain from, the instincts of a wide range of people so long as they are honestly dedicated to discovering the truth about natural phenomena.
I hope that what I have said so far goes part of the way to justify the use of model systems. I would like next to discuss briefly some of the properties of natural microbial ecosystems which seem to be worth incorporating in simpler models.
HOMOGENEITY VS. HETEROGENEITY
I have emphasized3,4 that microbiology has pursued the “homogeneity paradigm” for too long. Thus, the dictum that a mono culture in a stirred fermenter, preferably operating as a chemostat, is really all that is necessary to understand all the properties of microorganisms, seems to be a gross simplification. This is not in any way to deny the enormous importance of homogeneous systems and the chemostat in particular to studies in microbial ecology. The excellent chapter by Gottschal and Dijkhuisen in this volume (Chapter 2) illustrates both the power of the technique and the areas in microbial ecology where continuous culture has played such an important part. If the chemostat could answer all the questions concerning microbial behavior in their natural environments, however, there really would be little need for a book of this sort.
To recognize the need for more elaborate model systems is to accept a conceptual shift away from homogeneity to the heterogeneous systems that seem to predominate in nature. This conceptual shift is not merely to accept the fact implicit in the term “heterogeneity” that physico-chemical conditions alter as a function of position at a given time and of time at a given position, but it is to recognize and to accept instinctively the extreme importance of the space outside a cell to the growth and physiological behavior of that cell. However subtle and intricate and intrinsically fascinating are the ordered chemical reactions that go on within the cell envelope, they are dominated and modulated by the chemistry and physics of the regions surrounding it, yet this area has received only the scantiest attention in recent decades compared to the volumes published on the physiology and molecular biology of microbes.
A few of the factors affecting cell behavior include the distribution and flux of substrates needed for growth, the prevailing physical conditions of temperature, pressure, pH value, water potential ionic strength, etc., the presence of different phases and their physical behavior, the presence of inhibitory solutes, the presence of other prokaryotic and eukaryotic organisms, and the range of interactions that each exhibits. In microbiology, especially that of prokaryotic organisms, the business of living revolves around the distribution of solute molecules in a narrow aqueous layer surrounding the cytoplasmic membrane of the organism.
Once the importance of spatial heterogeneity is recognized, one is forced to accept the need for simple laboratory systems which incorporate heterogeneity factors. In the next section, I will briefly discuss a few spatially heterogeneous ecosystems, hopefully to illustrate just where laboratory models may have a useful part to play. This list is not intended to be exhaustive.
SOME SPATIALLY HETEROGENEOUS ECOSYSTEMS
Aquatic Systems
In general, water bodies have little spatial heterogeneity and many therefore behave like homogeneous fermenter systems. The oceans, seas, and large inland water bodies fall into this category at least at a “local” level. There are large-scale differences in chemistry that should be mentioned: for example, deep ocean troughts, some littoral regions, etc., can have differences in temperature, salinity, organic load, dissolved oxygen tension, and so on, while light penetration is a function of both of depth and of the clarity of the water. Related to gradients in light energy and spectrum is photosynthetic activity, which will in turn modulate the oxygen content of the waters. It may be that the ecology of such water bodies is best modeled in the laboratory by single-stage continuous culture systems running at very low concentrations of substrate. This is because although physico-chemical gradients may be present, they are extremely shallow, and this implies that interactions which depend on such gradients will be negligible in importance.
Rapidly flowing water bodies, including streams and “clean” shallow rivers, are also well mixed and substantially homogeneous. However, their ecology as a whole is often heterogeneous, since water flow is adjacent to solid surfaces which can support a substantial sessile population or to sediments which may be rich in microbial flora.
It should also be stressed that freely suspended individual cells are a comparative rarity in even these substantially homogeneou...
Inhaltsverzeichnis
Zitierstile für Revival: CRC Handbook of Laboratory Model Systems for Microbial Ecosystems, Volume I (1988)
APA 6 Citation
Wimpenny, J. (2019). CRC Handbook of Laboratory Model Systems for Microbial Ecosystems, Volume I (1st ed.). CRC Press. Retrieved from https://www.perlego.com/book/1501158/crc-handbook-of-laboratory-model-systems-for-microbial-ecosystems-volume-i-pdf (Original work published 2019)
Chicago Citation
Wimpenny, Julian. (2019) 2019. CRC Handbook of Laboratory Model Systems for Microbial Ecosystems, Volume I. 1st ed. CRC Press. https://www.perlego.com/book/1501158/crc-handbook-of-laboratory-model-systems-for-microbial-ecosystems-volume-i-pdf.
Harvard Citation
Wimpenny, J. (2019) CRC Handbook of Laboratory Model Systems for Microbial Ecosystems, Volume I. 1st edn. CRC Press. Available at: https://www.perlego.com/book/1501158/crc-handbook-of-laboratory-model-systems-for-microbial-ecosystems-volume-i-pdf (Accessed: 14 October 2022).
MLA 7 Citation
Wimpenny, Julian. CRC Handbook of Laboratory Model Systems for Microbial Ecosystems, Volume I. 1st ed. CRC Press, 2019. Web. 14 Oct. 2022.