
eBook - ePub
Laser Ultrasonics Techniques and Applications
L.E Drain
This is a test
Partager le livre
- 462 pages
- English
- ePUB (adapté aux mobiles)
- Disponible sur iOS et Android
eBook - ePub
Laser Ultrasonics Techniques and Applications
L.E Drain
Détails du livre
Aperçu du livre
Table des matiĂšres
Citations
Ă propos de ce livre
The first book devoted to laser techniques in the generation and reception of ultrasonic waves in materials, Laser Ultrasonics: Techniques and Applications provides a full description of the state of the art in all fields involving both lasers and ultrasonics. This practical book focuses mainly on the possible applications of the techniques, yet th
Foire aux questions
Comment puis-je résilier mon abonnement ?
Il vous suffit de vous rendre dans la section compte dans paramĂštres et de cliquer sur « RĂ©silier lâabonnement ». Câest aussi simple que cela ! Une fois que vous aurez rĂ©siliĂ© votre abonnement, il restera actif pour le reste de la pĂ©riode pour laquelle vous avez payĂ©. DĂ©couvrez-en plus ici.
Puis-je / comment puis-je télécharger des livres ?
Pour le moment, tous nos livres en format ePub adaptĂ©s aux mobiles peuvent ĂȘtre tĂ©lĂ©chargĂ©s via lâapplication. La plupart de nos PDF sont Ă©galement disponibles en tĂ©lĂ©chargement et les autres seront tĂ©lĂ©chargeables trĂšs prochainement. DĂ©couvrez-en plus ici.
Quelle est la différence entre les formules tarifaires ?
Les deux abonnements vous donnent un accĂšs complet Ă la bibliothĂšque et Ă toutes les fonctionnalitĂ©s de Perlego. Les seules diffĂ©rences sont les tarifs ainsi que la pĂ©riode dâabonnement : avec lâabonnement annuel, vous Ă©conomiserez environ 30 % par rapport Ă 12 mois dâabonnement mensuel.
Quâest-ce que Perlego ?
Nous sommes un service dâabonnement Ă des ouvrages universitaires en ligne, oĂč vous pouvez accĂ©der Ă toute une bibliothĂšque pour un prix infĂ©rieur Ă celui dâun seul livre par mois. Avec plus dâun million de livres sur plus de 1 000 sujets, nous avons ce quâil vous faut ! DĂ©couvrez-en plus ici.
Prenez-vous en charge la synthÚse vocale ?
Recherchez le symbole Ăcouter sur votre prochain livre pour voir si vous pouvez lâĂ©couter. Lâoutil Ăcouter lit le texte Ă haute voix pour vous, en surlignant le passage qui est en cours de lecture. Vous pouvez le mettre sur pause, lâaccĂ©lĂ©rer ou le ralentir. DĂ©couvrez-en plus ici.
Est-ce que Laser Ultrasonics Techniques and Applications est un PDF/ePUB en ligne ?
Oui, vous pouvez accĂ©der Ă Laser Ultrasonics Techniques and Applications par L.E Drain en format PDF et/ou ePUB ainsi quâĂ dâautres livres populaires dans Physical Sciences et Optics & Light. Nous disposons de plus dâun million dâouvrages Ă dĂ©couvrir dans notre catalogue.
Informations
1 Introduction
Since their invention in the early 1960s lasers have found applications in most areas of science and technology. Laser techniques are thus used in such diverse fields as spectroscopy, metrology, inspection, communications, fusion research, weapons systems and surgery. As a source of light the laser has a number of unique characteristics, which together make it a very versatile device. These include its ability to generate a well-collimated, intense beam of coherent light at a precisely defined wavelength. Lasers can be readily constructed to operate in continuous or pulsed mode (with pulse lengths from milliseconds down to picoseconds), and at wavelengths from infrared to ultraviolet. Not all the applications take advantage of all the features of the laser: some (e.g. laser welding) mainly require a high-intensity, well-collimated beam that can be accurately focused, while others (e.g. inter-ferometry) make use of the accurate wavelength and high coherence.
While the greatest initial impact of lasers was undoubtedly on optical techniques, they have more recently begun to make a significant contribution in fields such as ultrasonics. Ultrasonics has now become a broad and mature technology. Ultrasonic inspection methods are routinely used for the non-destructive examination of engineering materials and structures, ultrasonic diagnosis is routinely used in medicine, while ultrasonic measurements of displacement and dimension are widely used throughout industry. The combination of laser techniques with ultrasonics has led to some exciting new discoveries and applications. Before proceeding to consider the combination of lasers with ultrasonics, we briefly introduce the fields of ultrasonics and laser physics separately.
1.1 ULTRASONICS
Energy can be propagated through solids, liquids and gases as acoustic waves. In fluids there can in general only be one propagation mode, of longitudinal waves (i.e. displacements parallel to the direction of propagation) which take the form of alternate compressions and rarefactions; thus they are also known as compression(al) waves. Acoustic waves propagate with a velocity which is characteristic of the fluid medium. In elastic solids, which include most metals, the situation is more complicated. The medium can now accommodate transverse waves (displacements perpendicular to the direction of propagation) in addition to longitudinal waves (figure 1.1). The particle motions in what are known as elastic waves can in general be resolved into three perpendicular components, two transverse and one longitudinal. Each of the three modes has its own characteristic velocity, although in isotropic solids the two transverse velocities are equal (and also smaller than the longitudinal velocity).
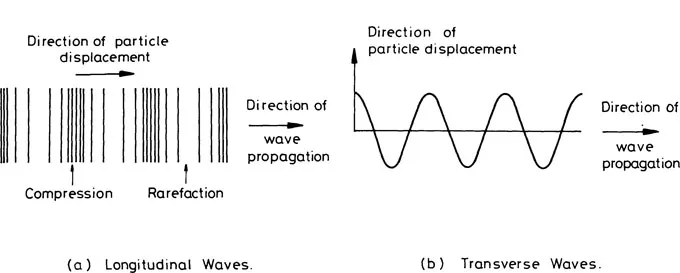
Figure 1.1 Schematic of (a) longitudinal and (b) transverse waves. In the latter case there is a plane of polarization defined in the plane of the page.
Acoustic waves (whether naturally or artificially generated) cover an extremely wide frequency spectrum. The audible range extends from about 50 Hz to a frequency between 12 and 20 kHz depending upon the age, etc, of the individual. Below this range lies infrasound, including most of the energy in earthquakes, for instance. Acoustic frequencies above about 20 kHz are known as ultrasound. Most commercial ultrasonics takes place within the range 50 kHzâ20 MHz, although higher frequency measurements are increasingly being made, to ~ 100 MHz. The more recent technique of acoustic (or ultrasonic) microscopy uses even higher frequencies, i.e. 200 MHzâ2 GHz. Gigahertz frequencies are currently the upper limit for ultrasonics. Above these frequencies there are quantized lattice vibrations (phonons). Phonon frequencies in solids typically extend up to 1013Hz. For present purposes we shall however restrict ourselves to a range below 109 Hz, which can in practice be treated by classical mechanics.
In common with all wave motions, the velocity of ultrasound is equal to the product of its wavelength and frequency. Thus for a typical longitudinal sound velocity in a solid of 5000 msâ1, the audible range extends over the wavelength range 0.25â100 m. At the lower end of the ultrasonic spectrum, the wavelengths in most solids are fractions of a metre, which still correspond to relatively large-scale vibrations. However, in the 1â5 MHz range (one of the most widely used in medical and industrial ultrasonics) the wavelengths are a few millimetres in metals and just less than a millimetre in water. Thus the waves are far more localized, and can probe the medium with much greater resolution. At a frequency of 2 GHz in the acoustic microscope the wavelength (in water) is 750 nm, comparable with that of visible light. Thermally excited phonon wavelengths may be as small as several interatomic spacings.
Ultrasound can be made to propagate relatively large distances in many solids and liquids. However, in addition to geometrical spreading, which takes the form of the inverse square law in three dimensions, there are a number of medium-specific loss mechanisms, such as absorption by the medium and scattering from discontinuities. All of these cause the signal to be attenuated. Although attenuation can have various origins, it generally increases as the frequency of the ultrasound increases. Thus, while ultrasound at tens or hundreds of kilohertz will propagate many metres in metals, these distances are typically reduced to centimetres much above 10 MHz. As gigahertz frequencies are approached, the waves will only propagate small fractions of a millimetre. Absorption and scattering similarly restrict the use of high-frequency ultrasound in liquids. In gases such as air the attenuation is extremely high and propagation paths of only a few centimetres may be attainable once the frequency rises much above 1 MHz.
In common with other wave motions, ultrasound undergoes various changes at boundaries, whether internal or external. When a beam of ultrasound impinges upon a boundary between two different fluid media, some of the energy will in general be transmitted through the boundary into the second medium, although refraction will occur. The rest of the energy will be reflected back from the boundary into the first medium. The proportions of ultrasonic energy transmitted and reflected can be calculated if the properties of the media are known. If the second medium is a gas such as air, then transmission into it can often be neglected because it is so small.
However, if either or both of the media are elastic solids, the situation becomes more complicated since solids can support three propagation modes. The conditions at the boundary ensure that in most cases at least two of the propagation modes couple together, so that energy can be transferred from compression to shear waves or vice versa. Mode conversion, as this is known, can be a benefit since potentially it enables more information to be extracted about the specimen under inspection. However, it also adds to the complexity of ultrasonic data and may lead to difficulties in interpretation.
The multiple reflections and mode conversions that occur at the boundaries of the specimen interfere with and reinforce one another to transfer energy into the normal modes of the specimen. These normal modes (specimen resonances) build up particularly quickly when the specimen is a regular solid such as a rectangular plate. These normal modes are generally avoided in ultrasonic measurements by methods such as time-gating (i.e. selecting a short time window) and band-pass filtering (the normal modes generally occur at lower frequencies).
Surfaces and interfaces are also of great interest for another reason: they are able to support various types of surface and interface wave. Surface waves are often detected with relatively large amplitude: this is because they only propagate over a two-dimensional surface rather than throughout three-dimensional space. Waves on rods and strips suffer even less attenuation due to geometrical spreading since they propagate in only one dimension. The most important surface wave is the Rayleigh wave. It causes points on the surface to move in an elliptical motion with (predominantly) perpendicular and parallel components. Also important are Lamb and other waves that couple together the motions on surfaces in close proximity. Energy can readily be coupled into interfacial waves from bulk waves (and vice versa) at boundaries.
From a theoretical viewpoint it is usually convenient to consider two main types of wave: plane waves and spherical waves. In the former case the source of the ultrasound is considered to be at infinity so that the wavefronts are parallel planes. Under these conditions the calculation of ultrasonic field strengths is usually simplified. The second most convenient ideal source is an infinitesimally small point. This generates spherical wavefronts. Whereas in the former case there is no attenuation due to geometrical spreading, in the latter the inverse square law applies, so that the intensity decays with the square of the distance from the point source. Regrettably the ultrasound from practical sources can only be approximated to either of these two cases.
The simplest model of a more realistic ultrasonic source is a vibrating piston of finite radius. Under continuous excitation the region immediately in front of the source receives waves from all points across the piston but after slightly different time delays. These waves thus interfere with one another to...