
eBook - ePub
Echocardiography in Pediatric and Congenital Heart Disease
From Fetus to Adult
Wyman W. Lai, Luc L. Mertens, Meryl S. Cohen, Tal Geva
This is a test
Partager le livre
- English
- ePUB (adapté aux mobiles)
- Disponible sur iOS et Android
eBook - ePub
Echocardiography in Pediatric and Congenital Heart Disease
From Fetus to Adult
Wyman W. Lai, Luc L. Mertens, Meryl S. Cohen, Tal Geva
Détails du livre
Aperçu du livre
Table des matiĂšres
Citations
Ă propos de ce livre
This comprehensive textbook on the echocardiographic assessment of pediatric and congenital heart disease has been updated for a second edition with an emphasis on new technologies. This highly-illustrated full-color reference contains over 1200 figures, and offers over 600 video clips on a companion website.
- Fully updated, with new chapters on the assessment of the post-Fontan procedure patient and on pregnancy and heart disease
- Each lesion chapter includes new section highlighting the key elements of the echocardiogram(s)
- Written by experts from the leading centers around the world, with numerous new authors
- Revision emphasizes new technologies and quality of images
- Comprehensive content contains overview of ultrasound physics, discussion of laboratory set-up, protocol for a standard pediatric echocardiogram and quantitative methods of echocardiographic evaluation, including assessment of diastolic function
- Also includes special techniques and topics including 3D echocardiography, intraoperative echocardiography, and fetal echocardiography
Foire aux questions
Comment puis-je résilier mon abonnement ?
Il vous suffit de vous rendre dans la section compte dans paramĂštres et de cliquer sur « RĂ©silier lâabonnement ». Câest aussi simple que cela ! Une fois que vous aurez rĂ©siliĂ© votre abonnement, il restera actif pour le reste de la pĂ©riode pour laquelle vous avez payĂ©. DĂ©couvrez-en plus ici.
Puis-je / comment puis-je télécharger des livres ?
Pour le moment, tous nos livres en format ePub adaptĂ©s aux mobiles peuvent ĂȘtre tĂ©lĂ©chargĂ©s via lâapplication. La plupart de nos PDF sont Ă©galement disponibles en tĂ©lĂ©chargement et les autres seront tĂ©lĂ©chargeables trĂšs prochainement. DĂ©couvrez-en plus ici.
Quelle est la différence entre les formules tarifaires ?
Les deux abonnements vous donnent un accĂšs complet Ă la bibliothĂšque et Ă toutes les fonctionnalitĂ©s de Perlego. Les seules diffĂ©rences sont les tarifs ainsi que la pĂ©riode dâabonnement : avec lâabonnement annuel, vous Ă©conomiserez environ 30 % par rapport Ă 12 mois dâabonnement mensuel.
Quâest-ce que Perlego ?
Nous sommes un service dâabonnement Ă des ouvrages universitaires en ligne, oĂč vous pouvez accĂ©der Ă toute une bibliothĂšque pour un prix infĂ©rieur Ă celui dâun seul livre par mois. Avec plus dâun million de livres sur plus de 1 000 sujets, nous avons ce quâil vous faut ! DĂ©couvrez-en plus ici.
Prenez-vous en charge la synthÚse vocale ?
Recherchez le symbole Ăcouter sur votre prochain livre pour voir si vous pouvez lâĂ©couter. Lâoutil Ăcouter lit le texte Ă haute voix pour vous, en surlignant le passage qui est en cours de lecture. Vous pouvez le mettre sur pause, lâaccĂ©lĂ©rer ou le ralentir. DĂ©couvrez-en plus ici.
Est-ce que Echocardiography in Pediatric and Congenital Heart Disease est un PDF/ePUB en ligne ?
Oui, vous pouvez accĂ©der Ă Echocardiography in Pediatric and Congenital Heart Disease par Wyman W. Lai, Luc L. Mertens, Meryl S. Cohen, Tal Geva en format PDF et/ou ePUB ainsi quâĂ dâautres livres populaires dans Medizin et Kardiologie. Nous disposons de plus dâun million dâouvrages Ă dĂ©couvrir dans notre catalogue.
Informations
PART I
Introduction to Cardiac Ultrasound Imaging
CHAPTER 1
Ultrasound Physics
Jan D'hooge1 and Luc L. Mertens2
1Department of Cardiovascular Sciences, Catholic University of Leuven; Medical Imaging Research Center, University Hospital Gasthuisberg, Leuven, Belgium
2Cardiology, The Hospital for Sick Children; Department of Pediatrics, University of Toronto, Toronto, ON, Canada
Physics and technology of echocardiography
Echocardiography is the discipline of medicine in which images of the heart are created by using ultrasound waves. Knowledge of the physics of ultrasound helps us to understand how the different ultrasound imaging modalities operate and also is important when operating an ultrasound machine to optimize the image acquisition.
This section describes the essential concepts of how ultrasound waves can be used to generate an image of the heart. Certain technological developments will also be discussed as well as how systems settings influence image characteristics. For more detailed treatment of ultrasound physics and imaging there is dedicated literature, to which readers should refer, for example [1, 2].
How the ultrasound image is created
The pulseâecho experiment
To illustrate how ultrasound imaging works, the acoustic âpulseâechoâ experiment can be used:
- A short electric pulse is applied to a piezoelectric crystal. This electric field will induce a shape change of the crystal through reorientation of its polar molecules. In other words, due to application of an electric field the crystal will momentarily deform.
- The deformation of the piezoelectric crystal induces a local compression of the tissue with which the crystal is in contact: that is, the superficial tissue layer is briefly compressed resulting in an increase in local pressure; this is the so-called acoustic pressure (Figure 1.1).
- Due to an interplay between tissue elasticity and inertia, this local tissue compression (with subsequent decompression, i.e., rarefaction) will propagate away from the piezoelectric crystal at a speed of approximately 1530 m/s in soft tissue (Figure 1.2). This is called the acoustic wave. The rate of compression/decompression determines the frequency of the wave and is typically 2.5â10 MHz (i.e., 2.5â10 million cycles per second) for diagnostic ultrasonic imaging. As these frequencies cannot be perceived by the human ear, these waves are said to be âultrasonic.â The spatial distance between subsequent compressions is called the wavelength (λ) and relates to the frequency (f) and sound velocity (c) as: λf = c. During propagation, acoustic energy is lost mostly as a result of viscosity (i.e., friction) resulting in a reduction in amplitude of the wave with propagation distance. The shorter the wavelength (i.e., the higher the frequency), the faster the particle motion and the larger the viscous effects. Higher frequency waves will thus attenuate more and penetrate less deep into the tissue.
- Spatial changes in tissue density or tissue elasticity will result in a disturbance of the propagating compression (i.e., acoustic) wave and will cause part of the energy in the wave to be reflected. These so-called âspecular reflectionsâ occur, for example, at the interface between different types of tissue (e.g., blood and myocardium) and behave in a similar way to optic waves in that the direction of the reflected wave is determined by the angle between the reflecting surface and the incident wave (cf. reflection of optic waves on a water surface). When the spatial dimensions of the changes in density or compressibility become small relative to the wavelength (i.e., below âŒ100 ÎŒm), these inhomogeneities will cause part of the energy in the wave to be scattered, that is, retransmitted in all possible directions. The part of the scattered energy that is retransmitted back into the direction of origin of the wave is called backscatter. Both the specular and backscattered reflections propagate back towards the piezoelectric crystal.
- When the reflected (compression) waves impinge upon the piezoelectric crystal, the crystal deforms. This results in relative motion of its (polar) molecules and generation of an electric field, which can be detected and measured. The amplitude of this electric signal is directly proportional to the amount of compression of the crystal, that is, the amplitude of the reflected/backscattered wave. This electric signal is called the radio-frequency (RF) signal and represents the amplitude of the reflected ultrasound wave as a function of time (Figure 1.3). Because reflections occurring further away from the transducer need to propagate further, they will be received later. As such, the time axis in Figure 1.3 can be replaced by the propagation distance of the wave (i.e., depth). The signal detected by the transducer is typically electronically amplified. The amount of amplification has a preset value but can be modified on an ultrasound system by using the âgainâ button (typically the largest button on the operating panel). Importantly, the overall gain will amplify both the signal and potential measurement noise and will thus not affect the signal-to-noise ratio.
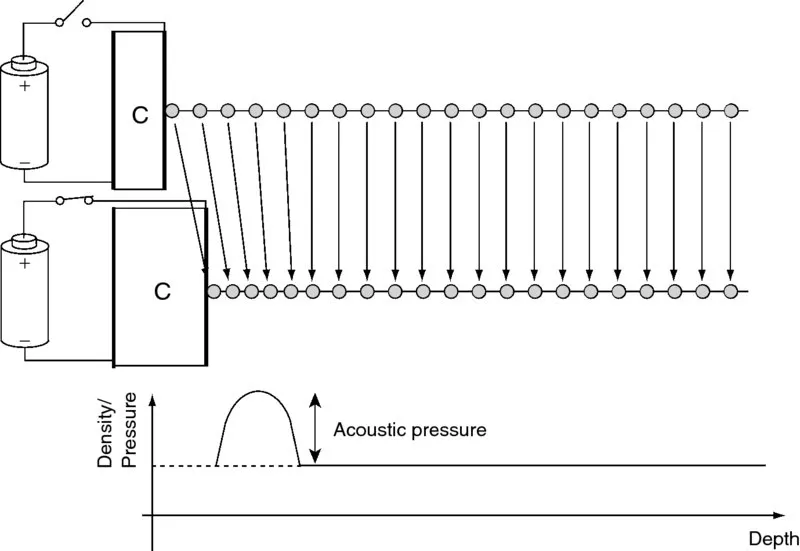
Figure 1.1 Local tissue compression due to deformation of the piezoelectric crystal when applying an electric field.
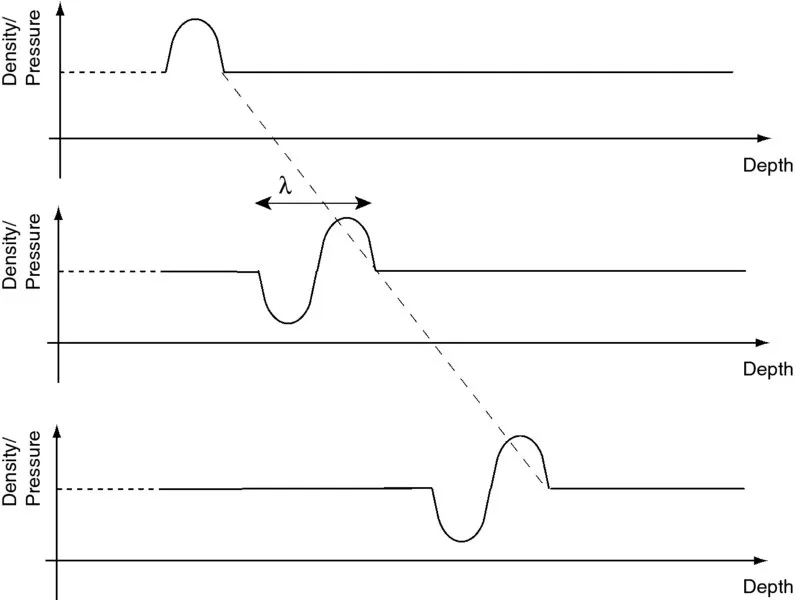
Figure 1.2 The local tissue compression/ decompression propagates away from its source at a speed of approximately 1530 m/s in soft tissue.
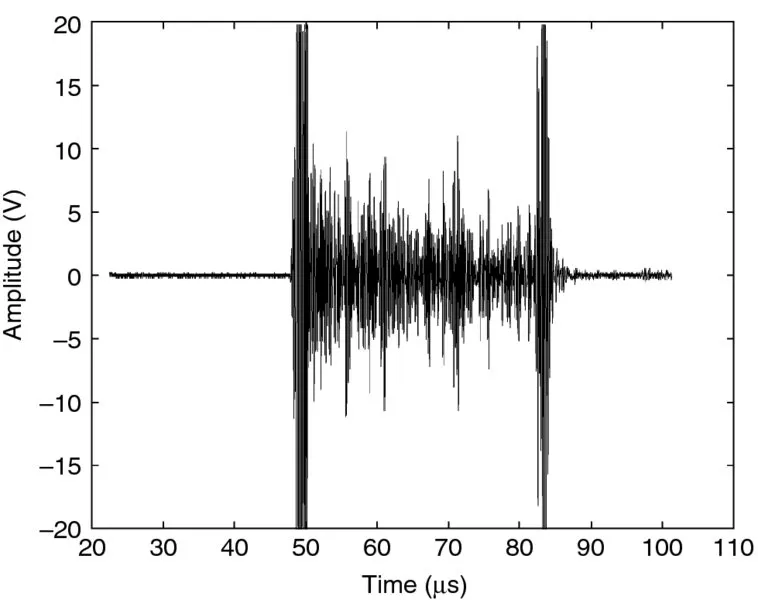
Figure 1.3 The reflected amplitude of the reflected ultrasound waves as a function of time after transmission of the ultrasound pulse is called the radio-frequency (RF) signal.
In the example shown in Figure 1.3, taken from a water tank experiment, two strong specular reflections can be observed (around 50 and 82 ÎŒs, respectively) while the lower amplitude reflections in between are scatter reflections. In clinical echocardiography, the most obvious specular reflection is the strong reflection coming from the pericardium observed in the parasternal views as a consequence of its increased stiffness with respect to the surrounding tissues. The direction of propagation of the specular reflection is determined by the angle between the incident wave and the reflecting surface. Thus, the strength of the observed reflection will depend strongly on the exac...